|
ENVIS Technical Report 77, Febraury 2014 |
ENVIRONMENT MONITORING IN THE NEIGHBOURHOOD
|
PROTOCOL FOR WATER QUALITY MONITORING (PHYSICAL, CHEMICAL AND BIOLOGICAL PARAMETERS)
|
WATER SAMPLING |
Sampling:
The sample collected should be small in volume, enough to accurately represent the whole water body. The water sample tends to modify itself to the new environment. It is necessary to ensure that no significant changes occur in the sample and preserve its integrity till analysed (by retaining the same concentration of all the components as in the water body). The essential objectives of water quality assessment are to:
- Define the status and trends in water quality of a given water body.
- Analyse the causes for the observed conditions and trends.
- Identify the area specific problems of water quality and provide assessments in the form of management to evaluate alternatives that help in decision-making.
|
Site selection in a waterbody: Sampling sites for the water body/lake are selected to represent the water quality at different points and depths. Generally three sampling sites are selected for monitoring.
- Inlet: the point where the principal feeder opens into the lake.
- Center: the point that gives the general water quality of the lake.
- Outlet: the place where the overflow occurs.
|
Types of sampling: Generally three types of sampling are adopted for collecting water samples.
- Grab or Catch sampling: the sample is collected at a particular time and place that represents the composition of the source at that particular point and time.
- Composite sampling: a mixture of grab samples is collected at the same sampling point at different time intervals.
- Integrated sampling:a mixture of grab samples collected at different points simultaneously.
|
Sampling frequency
The quality of water varies with time in a water body due to various natural and human induced factors. The monitoring has to be done in a way that records all the changes in the quality. The sampling frequencies generally adopted in monitoring are:
- Monthly sampling covering all seasons (preferably for 24 months).
- Weekly sampling for one year.
- Consecutive day sampling during the study period.
- Hourly sampling for 24 hours (example: parameters such as dissolved oxygen, pH, etc.).
|
Variations in water quality are mainly due to changes in the concentrations of the components of the water flowing into the water body. These variations can be man-made or natural and can either be cyclic or random.
- Random variations: due to spasmodic, often unpredictable events such as accidental oil spills, sewage leaks, overflows, etc.
- Cyclic variations: may be a result of regular seasonal changes triggering certain natural processes such as rainfall, snowmelts and seasonal temperature changes, altering the ecosystem. Seasonal growth and decay of vegetation will also rise due to cyclic changes in the composition of water.
In lakes, the mass of water and good lateral mixing provide inertia against any rapid modifications due to inputs and outputs. |
Sampling container: The sampling container should not react with the sample, be of adequate capacity to store the sample and be free from contamination. |
Sampling method: Grab sampling can be performed at the inlet, center and outlet in most of the water bodies studied to assess their physical and chemical qualities. The samples can be collected in thoroughly cleaned 2.5-litre inert plastic containers, which is rinsed with distilled and lake/tank water before collection.
Note: Water samples were collected in a sampling bottle avoiding floating materials. The stoppers of the sample containers were closed properly to prevent outside contamination. The container was labelled describing the name of the water body, date, time, sampling-point, and conditions under which it was sampled. |
Analyses of Physical, Chemical and Biological Parameters |
The parameters analysed to assess the water quality are broadly divided into:
- Physical parameters: Colour, Temperature, Transparency, Turbidity and Odour.
- Chemical parameters: pH, Electrical Conductivity (E.C), Total Solids (TS), Total Dissolved Solids (TDS), Total Suspended Solids (TSS), Total Hardness, Calcium Hardness, Magnesium Hardness, Nitrates, Phosphates, Chlorides, Dissolved Oxygen (D.O), Biological Oxygen Demand (BOD), Chemical Oxygen Demand (COD), Fluorides, Free Carbon-dioxide, Potassium and Sodium.
- Biological parameters: The biological parameters involved the qualitative analyses of planktons
|
Field measurement: The field (on-spot) parameters measured include pH, conductivity, dissolved oxygen, temperature and transparency. |
Sample Preservation: Between the time a sample is collected and analysed in the laboratory, physical, chemical and biochemical reactions may take place in the sample container leading to changes in the intrinsic quality of the sample, making it necessary to prevent or minimize these changes with suitable preservatives such as alcohol and mercuric chloride. Highly unstable parameters such as pH, temperature, transparency, free carbon-dioxide, dissolved oxygen, etc. is measured at the sampling site. The preservation procedure includes keeping the samples in the dark, adding chemical preservative, lowering the temperature to retard reactions, or combinations of these. |
Experiment |
Preservative |
Max. holding time |
BOD |
Cool, 4o C |
4 hours |
Calcium |
Cool, 4o C |
7 days |
Chloride |
Cool, 4o C |
7 days |
COD |
Cool, 4o C |
24 hours |
Dissolved Oxygen* |
Fix on site |
6 hours |
Magnesium |
Cool, 4o C |
7 days |
Nitrate + Nitrite |
Cool, 4o C |
24 hours |
pH |
None |
6 hours |
Phosphorus* |
|
|
Dissolved |
Filter on site using 0.45µm filter |
24 hours |
Inorganic |
Cool, 4o C |
24 hours |
Ortho |
Cool, 4o C |
24 hours |
Total |
Cool, 4o C |
1 month |
Potassium |
Cool, 4o C |
7 days |
Specific Conductance |
Cool, 4o C |
24 hours |
Sodium |
Cool, 4o C |
7 days |
(Source: Analytical Methods Manual, Water quality branch, Environment Canada, 1981) |
WATER TEMPERATURE |
1 |
WATER TEMPERATURE: The water temperature is important for its effects on the chemistry, and biological reactions in the organisms in water. A rise in temperature of the water leads to the speeding up of the chemical reactions in water, reduces the solubility of gases, affects carbon dioxide-carbonate-bicarbonate equilibrium and amplifies the tastes and odours. Temperature influences water chemistry, e.g. DO, solubility, density, pH, alkalinity, salinity, conductivity etc. At elevated temperatures, metabolic activity of organism’s increases, requiring more oxygen but at the same time the solubility of oxygen decreases. Water temperature is important in relation to fish life. The disease resistance in the fishes also decreases with rise in temperature. The aquatic organisms show varied sensitivities to temperature. The temperature of drinking water has an influence on its taste. |
Procedure
Clean the pH-TDS-EC probe/thermometer (Hg bulb region) with distilled water and fill the container with water sample. Then check the water temperature of the sample by dipping the pH-TDS-EC probe into sample and record it in degrees Celsius (ºC). |
Apparatus: pH-TDS-EC probe/ Laboratory thermometer, Beaker |
Do’s and Dont’s:
- Clean the electrode (sensor parts) of the meter with distilled water and wipe properly using tissue paper, before and after usage.
- Calibrate the meters prior to each field trip. (Follow internal calibration solutions as per the Company instructions)
- Ensure: Company instructions for field use, maintenance and storage of the pH-TDS-EC probe have been followed properly.
- Note down the temperature readings of each sample.
- Don’t expose the meter/laboratory thermometer directly to sunlight.
|
Results |
Date |
S1 |
S2 |
S3 |
|
Limits (Inland) |
|
|
|
|
|
|
Inference |
|
References |
APHA (1998); Trivedi R. K. and Goel P. K. (1986); Ramachandra T. V. and Ahalya N. (2001) |
pH: pH is the measure of the acid-base balance of a solution and is defined as the negative logarithm to the base 10 of the hydrogen ion (H+) concentration. The pH scale runs from 0 to 14 (i.e., very acidic to very alkaline) with pH 7 representing a neutral solution. When the pH is less than 7, it is said to be acidic; a pH greater than 7 is basic.
pH tends to increase during day largely due to the photosynthetic activity (consumption of carbondioxide) and decreases during night due to respiratory activity. pH is also governed by the equilibrium between carbon dioxide/bicarbonate/carbonate ions. The change in the intensity of acidity or alkalinity of an aquatic ecosystem can be due to inflow of industrial effluents, domestic sewage and atmospheric deposition of acid-forming substances. Diurnal variations in pH can take place due to photosynthesis and respiration cycles of algae in eutrophic waters. Acidity of water is controlled by strong mineral acids, weak acids (such as carbonic, humic and fulvic acids) and hydrolysing salts of metals such as iron and aluminium. Waste water and polluted natural waters have pH values lower or higher than 7 based on the nature of the pollutant. Changes in pH endanger the lives of the organisms in the water.
A lower pH value, below 4 will produce sour taste whereas pH above 8.5 produces an alkaline taste. If the pH is below 6.5, corrosion of pipes occur, thus toxic metals like Zinc, Lead, Cadmium and Copper etc. are released. Higher pH values induce scale formation in water heating apparatus, production of toxic trihalomethanes and also reduce the germicidal potential of chlorine. |
Procedure
- Colorimetric method: About 10ml of the sample is taken in a wide mouth test tube, 0.2 ml of BDH indicator is added, and shaken gently. The colour developed is matched with the chart and the pH noted.
- Electrometric method: The pH is determined by measuring the Electro Motive Force (E.M.F) of a cell comprising an indicator electrode (an electrode responsive to hydrogen ions such as a glass electrode) immersed in the test solution and the reference electrode (usually a mercury/calomel electrode). Contact between the test solution and the reference electrode is usually got by means of a liquid junction, which forms a part of reference electrode. E.M.F of this cell is measured with pH meter that is a high impedance voltmeter calibrated in terms of pH. The electrode is allowed to stand for 2 minutes to stabilize before taking reading for reproducible results (at least ±0.1 pH units).
|
Apparatus:
- pH indicator (BDH) method: BDH Indicator (Universal Indicator) and test tubes.
- Electrometric method: Glass electrode, reference electrode (mercury/calomel or silver/silver chloride) and pH meter.
|
Do’s and Dont’s:
- Clean the meters with distilled water and wipe properly using tissue paper, before and after usage.
- Calibrate the meters prior to each field trip.
- Ensure that all the instructions on use, maintenance and storage of the pH-TDS-EC probe have been followed.
- Note down the pH readings of each sample.
|
Results |
Date |
S1 |
S2 |
S3 |
|
Limits (Inland) |
|
|
|
|
|
Permissible
7-8.5
Excessive
Not less than 6.5 or greater than 9.2 |
Inference |
|
References |
APHA (1998); Trivedi R. K. and Goel P. K. (1986); Ramachandra T. V. and Ahalya N. (2001) |
ELECTRICAL CONDUCTIVITY (EC) |
3 |
ELECTRICAL CONDUCTIVITY: Conductivity denotes the ability of an aqueous solution to conduct an electrical current. It depends on the presence of ions, their total concentration, mobility, valence, relative concentration and on the temperature of measurement. Electrolytes in a solution dissociate into positive (cations) and negative (anions) ions and impart conductivity. Solution of most inorganic acids, bases and salts (chloride, nitrate, sulfate, phosphate anions, sodium, magnesium, calcium, iron, and aluminium cations) are relatively good conductors unlike organic compounds (oil, phenol, alcohol, and sugar). Conductivity increases with temperature.
Conductance is defined as the reciprocal of the resistance involved and expressed as mho or Siemen (s).
G=1/R [where, G – Conductance (mho or Siemens) and R – Resistance]
The amount of current that can flow through a solution is proportional to the concentration of dissolved ionic species in the solution. Thus, higher the concentration of electrolytes in water, the more is its electrical conductivity. i.e. lesser the resistance. |
Procedure
The electrode of the conductivity meter is dipped into the sample, and the readings are noted for stable value shown as mS/cm. |
Apparatus: Conductivity meter, 100ml Beaker |
Do’s and Dont’s:
- Clean the meters with distilled water and wipe properly using tissue paper, before and after usage.
- Calibrate the meters prior to each field trip.
- Ensure that all the instructions on use, maintenance and storage of the pH-TDS-EC probe has been followed.
- Note down the EC readings of each sample.
|
Results |
Date |
S1 |
S2 |
S3 |
|
Limits (Inland) |
|
|
|
|
|
Permissible
750-2000
Excessive
>2000 |
Inference |
|
References |
APHA (1998); Trivedi R. K. and Goel P. K. (1986); Ramachandra T. V. and Ahalya N. (2001) |
TOTAL DISSOLVED SOLIDS (TDS) |
4 |
TDS : The substances that would pass through the filter paper but will remain as residue when the water evaporates which includes dissolved minerals and salts, humic, tannin and pyrogens. A constant level of minerals in the water is necessary for aquatic life. Changes in the amounts of dissolved solids can be harmful because the density of total dissolved solids determines the flow of water in and out of an organism’s cells. Many of these dissolved solids contain chemicals, such as nitrogen, phosphorous, and sulfur, which are the building blocks of molecules for life. Concentration of total dissolved solids that are too high or too low may limit the growth and may lead to the death of many aquatic organisms. High concentrations of total dissolved solids may reduce water clarity, which contributes to a decrease in photosynthesis and lead to an increase in water temperature. Many aquatic organisms cannot survive in high temperatures. The conductivity in water is affected by the presence of inorganic dissolved solids such as chloride, nitrate, sulfate, and phosphate anions or sodium, magnesium, calcium, iron, and aluminum cations. These particles contribute the dissolved solids of the water. Waters with high dissolved solids generally are of inferior palatability and may induce an unfavourable physiological reaction. |
Procedure
Clean the pH-TDS-EC probe with distilled water and fill the container with water sample. Then the total dissolved solids is determined by dipping the pH-TDS-EC probe into sample and express in parts per million (ppm). |
Apparatus: pH-TDS-EC probe, 100ml Beaker |
Do’s and Dont’s:
- Clean the meters with distilled water and wipe properly using tissue paper, before and after usage.
- Calibrate the meters prior to each field trip.
- Ensure that all the instructions on use, maintenance and storage of the pH-TDS-EC probe has been followed.
- Note down the TDS readings of each sample.
|
Results |
Date |
S1 |
S2 |
S3 |
|
Limits (Inland) |
|
|
|
|
|
Permissible
500
Excessive
1500 |
Inference |
|
References |
APHA (1998); Trivedi R. K. and Goel P. K. (1986); Ramachandra T. V. and Ahalya N. (2001) |
TRANSPARENCY (LIGHT PENETRATION) |
5 |
TRANSPARENCY: Solar radiation is the major source of light energy in an aquatic system, governing the primary productivity. Transparency is a characteristic of water that varies with the combined effect of colour and turbidity. It measures the light penetrating through the water body and is determined using Secchi disc. |
Procedure
Transparency is measured by gradually lowering the Secchi disc at respective sampling points. The depth at which it disappears in the water (X1) and reappears (X2) is noted.
The transparency of the water body is computed as follows:
Transparency (Secchi Disc Transparency) = (X1 + X2 )/2
Where, X1 = Depth at which Secchi disc disappears
X2 = Depth at which Secchi disc reappears
|
Apparatus: Secchi disc, a metallic disc of 20cm diameter with four quadrats of alternate black and white on the upper surface. The disc with centrally placed weight at the lower surface, is suspended with a graduated cord at the center.
|
Do’s and Dont’s:
- Clean the secchi disc before and after usage.
- Handle the secchi disc with care.
- Note down the readings of each sample.
|
Results |
Date |
S1 |
S2 |
S3 |
|
Limits (Inland) |
|
|
|
|
|
Permissible
Excessive |
Inference |
|
References |
APHA (1998); Trivedi R. K. and Goel P. K. (1986); Ramachandra T. V. and Ahalya N. (2001) |
TURBIDITY |
6 |
TURBIDITY: Turbidity is an expression of optical property; wherein light is scattered by suspended particles present in water (Tyndall effect) and is measured using a nephelometer. Any substance having a particle size more than 10‑9 will produce turbidity in water. Suspended and colloidal matter such as clay, silt, finely divided organic and inorganic matter; plankton and other microscopic organisms cause turbidity in water. Turbidity affects light scattering, absorption properties and appearance in a water body. Increase in the intensity of scattered light results in higher values of turbidity. Turbidity makes the water unfit for domestic purposes, food and beverage industries, and many other industrial purposes. In natural waters, turbidity restricts light penetration for photosynthesis.
Nephelometric measurement is based on comparison of the intensity of scattered light of the sample with the intensity of light scattered by a standard reference suspension (Formazin polymer) under similar conditions. |
Procedure: The nephelometer is calibrated using distilled water (Zero NTU) and a standard turbidity suspension of 40 NTU. The thoroughly shaken sample is taken in the nephelometric tube and the value is recorded.
Turbidity (NTU) = (Nephelometer readings) (Dilution factor*)
* If the turbidity of the sample is more than 40 NTU, then the sample is diluted and the dilution factor is accounted in final calculations. |
Apparatus:Nephelometer (It detects scattered light at 90o to the incident beam of light. It consists of a light source for illuminating the sample. One or more photoelectric detectors with a display unit indicate the intensity of light scattered at 90o to the path of incident light.), sample cells, lab-glass wares. |
Do’s and Dont’s:
- Collect the sample directly into the sampling cuvet (cell).
- Do not leave the sample cell in the meter so as to avoid scratches.
- Empty the sample at each site and rinse properly.
- Note down the Turbidity readings of each sample.
- Wipe the cell properly with tissue paper and ensure that the outside of the cell is clean and dry before placing the cell in to the meter.
|
Results |
Date |
S1 |
S2 |
S3 |
|
Limits (Inland) |
|
|
|
|
|
Permissible
5 NTU
Excessive
25 NTU |
Inference |
|
References |
APHA (1998); Trivedi R. K. and Goel P. K. (1986); Ramachandra T. V. and Ahalya N. (2001) |
DISSOLVED OXYGEN (DO) |
7 |
DISSOLVED OXYGEN: Oxygen is essential to all forms of aquatic life. DO depend upon the physical, chemical and biochemical activities in the water body. The analysis of DO is a key test in water pollution and waste treatment control. The two main sources of dissolved oxygen are diffusion of oxygen from the air and photosynthetic activity. Low levels of DO frequently indicate a high concentration of decaying organic matter in the water. As bacteria digest organic matter, they use up oxygen, leaving little for the other aquatic creatures. The factors affecting the DO are (1) temperature: as temperature increases, the amount of oxygen (or any gas) dissolved in water decreases. (2) Partial pressure of O2 in contact with water: at high altitudes, less oxygen is dissolved in water because the partial pressure of oxygen in the atmospheric is low (3) Salinity: the solubility of gases O2 and CO2 decreases with increasing salinity.
D.O (mg/L) |
Water quality |
Above 8.0 |
Good |
6.5-8.0 |
Slightly polluted |
4.5-6.5 |
Moderately polluted |
4.0-4.5 |
Heavily polluted |
Below 4.0 |
Severely polluted |
Winkler’s method
Principle: Oxygen present in the sample oxidizes the dispersed divalent manganous hydroxide to the higher valency to precipitate as a brown hydrated oxide after addition of potassium iodide and sodium hydroxide. Upon acidification, manganese reverts to its divalent state and liberates iodine from potassium iodide, equivalent to the original dissolved oxygen content of the sample. The liberated iodine is titrated against N/80 sodium thiosulphate using fresh iodine as an indicator. |
Calculation: NIVI = N2V2 (Molar equivalence formula)
(ml*N) of sodium thiosulphate * 8 * 1000
Dissolved oxygen, mg/L =
V2 [(V1 - V)/V1]
Where, V1 = Volume of sample bottle
V2 = Volume of contents titrated
V = Volume of MnSO4 and KI added (2ml)
Procedure
The samples are collected in BOD bottles, to which 1ml of manganous sulphate and 1ml of potassium iodide are added and sealed. This is mixed well and the precipitate is allowed to settle down. Then, 1ml of concentrated sulphuric acid is added, and mixed well until all the precipitate dissolves. 25 ml of the sample is measured into the conical flask and titrated against 0.025N sodium thiosulphate using starch as an indicator. The end point is the change of colour from blue to colourless. |
Apparatus: BOD bottle-125ml, measuring cylinder, conical flask, 1ml glass pipette etc. |
Reagents:
Manganous sulphate solution: Dissolved 100 g of manganous sulphate in 200ml of distilled water and heated to dissolve salt and filtered after cooling.
- Alkaline potassium iodide solution: Dissolved 100 g of KOH and 50 g of KI in 200ml of pre-boiled distilled water.
- Starch indicator:1g of starch is dissolved in 100 ml of warm distilled water and added few drops of formaldehyde solution.
- Stock sodium thiosulphate (0.1 N):24.82g of sodium thiosulphate pentahydrate (Na2S202. 5H2O) is dissolved in distilled water and made up to 1000ml.
- Standard sodium thiosulphate (0.025N): 250ml of the stock sodium thiosulphate pentahydrate is made up to 1000ml with distilled water to give 0.025N.
|
Do’s and Dont’s
- Label the BOD bottle with waterproof pen before collecting sample.
- Ensure that no air bubble enters the BOD bottle while filling it with water sample.
- Clean and different microtips should be used for each reagent.
- Handle the reagents and acids with extreme care. Wear disposable gloves if possible.
- Don’t keep the BOD under direct sunlight while doing experiment as it will interfere with the results.
|
Results |
Date |
S1 |
S2 |
S3 |
|
Limits (Inland) |
|
|
|
|
|
Permissible
3
Excessive
6 |
Inference |
|
References |
APHA (1998); Trivedi R. K. and Goel P. K. (1986); Ramachandra T. V. and Ahalya N. (2001) |
BIOLOGICAL OXYGEN DEMAND (BOD) |
8 |
BIOLOGICAL OXYGEN DEMAND: BOD is the amount of oxygen required by microorganisms for stabilizing biologically decomposable organic matter (carbonaceous) in water under aerobic conditions. The test is used to determine the pollution load of wastewater, the degree of pollution and the efficiency of wastewater treatment methods. 5-Day BOD test being a bioassay procedure (involving measurement of oxygen consumed by bacteria for degrading the organic matter under aerobic conditions) requires the addition of nutrients and maintaining the standard conditions of pH and temperature and absence of microbial growth inhibiting substances.
The method consists of filling the samples in airtight bottles of specified size and incubating them at specified temperature (20 oC) for 5 days. The difference in the dissolved oxygen measured initially and after incubation gives the BOD of the sample.
Calculation:
BOD, mg/L = (D0 – D5) x Dilution factor
Where,
D0 = Initial DO in the sample
D5 = DO of the sample after 5 days |
|
Procedure
The sample having a pH of 7 is determined for first day DO. Various dilutions (at least 3) are prepared to obtain about 50% depletion of D.O. using sample and dilution water. The samples are incubated at 20 oC for 5 days and the 5th day D.O is noted using the Winkler’s method. A reagent blank is also prepared in a similar manner. |
Apparatus: BOD bottles - 125ml capacity, air incubator - to be controlled at 20 oC ± 1 oC, magnetic stirrer. |
Reagents:
- Manganous sulphate solution: Dissolved 100 g of manganous sulphate in 200ml of distilled water and heated to dissolve salt and filtered after cooling.
- Alkaline potassium iodide solution: Dissolved 100 g of KOH and 50 g of KI in 200ml of pre-boiled distilled water.
- Starch indicator:1g of starch is dissolved in 100 ml of warm distilled water.
- Stock sodium thiosulphate (0.1 N):24.82g of sodium thiosulphate pentahydrate (Na2S2O2. 5H2O) is dissolved in distilled water and made up to 1000ml.
- Standard sodium thiosulphate (0.025N): 250ml of the stock sodium thiosulphate pentahydrate is made up to 1000ml with distilled water to give 0.025N.
- Formaldehyde: preservative for freshly prepared starch solution
|
Do’s and Dont’s
- Rinse the BOD bottles properly with distilled water before initiating the experiment.
- Label the BOD bottle with waterproof labels/pen before collecting sample.
- Ensure that no air bubble enters the BOD bottle while filling it with water sample.
- Handle the reagents and acids with extreme care. Wear disposable gloves if possible.
- Don’t keep the BOD bottles under direct sunlight while doing experiment as it will interfere with the results.
*Alkaline KI – potential skin irritant
*Conc. H2SO4 – corrosive acid |
Results |
Date |
S1 |
S2 |
S3 |
|
Limits (Inland) |
|
|
|
|
|
Permissible 10
Excessive 30 |
Inference |
|
References |
APHA (1998); Trivedi R. K. and Goel P. K. (1986); Ramachandra T. V. and Ahalya N. (2001) |
CHEMICAL OXYGEN DEMAND (COD) |
9 |
CHEMICAL OXYGEN DEMAND: COD is the measure of oxygen equivalent to the organic content of the sample that is susceptible to oxidation by a strong chemical oxidant. The intrinsic limitation of the test lies in its ability to differentiate between the biologically oxidisable and inert material. It is measured by the open reflux method.
The organic matter in the sample gets oxidized completely by strong oxidizing agents such as potassium dichromate in the presence of conc. sulphuric acid to produce carbon-dioxide and water. The excess potassium dichromate remaining after the reaction is titrated with Ferrous Ammonium Sulphate (FAS) using ferroin indicator to determine the COD. The dichromate consumed gives the oxygen required for the oxidation of the organic matter. |
Procedure
15ml of conc. sulphuric acid with 0.3g of mercuric sulphate and a pinch of silver sulphate along with 5ml of 0.025M potassium dichromate is taken into a Nessler's tube. 10ml of sample (thoroughly shaken) is pipetted out into this mixture and kept for about 90 minutes on the hot plate for digestion. 40ml of distilled water is added to the cooled mixture (to make up to 50ml) and titrated against 0.25M FAS using ferroin indicator, till the colour turns from blue green to wine red indicating the end point. A reagent blank is also carried out using 10ml of distilled water.
Calculation:
COD, mg/L = (b-a) x N of FAS x 1000 x 8
ml of sample
Where,
a = ml of titrant with sample.
b = ml of titrant with blank. |
Apparatus: Reflux apparatus, Nessler’s tube, Erlenmeyer flasks, hot plate and lab glassware. |
Reagents:
- Standard potassium dichromate solution (0.250M): 12.25g of potassium dichromate dried at 103 oC for about 2 hours is dissolved in distilled water and made up to 1000ml.
- Standard ferrous ammonium sulphate (FAS) 0.25N:98g of FAS is dissolved in minimum distilled water to which 20ml of conc. sulphuric acid is added and made up to 1000ml using distilled water to give 0.25N of ferrous ammonium sulphate.
- Ferroin indicator:1.485g of 1,10-Phenanthroline monohydrate and 695mg of ferrous sulphate is dissolved in 100ml of distilled water.
- Conc. sulphuric acid
- Silver sulphate crystal
- Mercuric sulphate crystals
|
Do’s and Dont’s
- Label the cleaned Erlenmeyer flasks properly.
.
- Handle the reagents and acids with extreme care.
- It is safe to wear gloves.
|
Results |
Date |
S1 |
S2 |
S3 |
|
Limits (Inland) |
|
|
|
|
|
Permissible
30
Excessive
100 |
Inference |
|
References |
APHA (1998); Trivedi R. K. and Goel P. K. (1986); Ramachandra T. V. and Ahalya N. (2001) |
FREE CARBON-DIOXIDE |
10 |
FREE CARBON-DIOXIDE: The important source of free carbon-dioxide in surface water bodies is mainly respiration and decomposition by aquatic organisms. It reacts with water partly to form calcium bicarbonate and in the absence of bicarbonates gets converted to carbonates releasing carbon-dioxide. |
Procedure
A known volume (25ml) of the sample was measured into a conical flask. 2-3 drops of phenolphthalein indicator was added and titrated against 0.22N sodium hydroxide till the pink colour persisted indicating the end point.
If the pink colour appears on adding phenolphthalein it indicates the absence of free carbon-dioxide. |
Apparatus: Lab glassware - measuring cylinder, pipette, conical flask, etc. |
Reagents:
- Sodium hydroxide solution (0.22N):1g of sodium hydroxide was dissolved in 100ml of distilled water and made up to 1000ml to give 0.22N.
- Phenolpthalein indicator: Dissolve 0.5 g of phenolphthalein in 50 ml of 95% ethanol and add 50 ml of distilled water. Add 0.05 N CO2 free NaOH solution dropwise, until the solution turns faintly pink.
|
Do’s and Dont’s
- Ensure that the Erlenmeyer flasks to be used are washed properly and labeled.
- Handle the reagents and acids with extreme care.
|
Results |
Date |
S1 |
S2 |
S3 |
|
Limits (Inland) |
|
|
|
|
|
Permissible
Excessive |
Inference |
|
References |
APHA (1998); Trivedi R. K. and Goel P. K. (1986); Ramachandra T. V. and Ahalya N. (2001) |
ALKALINITY |
11 |
ALKALINITY: In natural water, that are not highly polluted alkalinity is more commonly found than acidity. Alkalinity is the good indicator of presence of dissolved inorganic carbon (bicarbonates and carbonate anions). Some of the minor contributions to alkalinity come from ammonia, phosphates, borates, silicates and other basic substances.
Alkalinity is beneficial to aquatic animals and plants, because it buffers both the natural and human induced pH changes. Water with high alkalinity generally have a high concentration of dissolved inorganic carbon (in the form of HCO3- and CO32-), which can be converted to biomass by photosynthesis.
Titrating a basic water sample with acid to pH 8.3 measures phenolphthalein alkalinity. Phenolphthalein alkalinity primarily measures the amount of carbonate ion (CO32-) present in the sample. Titrating with acid to pH 3.7 measures methyl orange alkalinity or total alkalinity. Total alkalinity measures the neutralizing effects of all the bases present.
Calculate total phenolphthalein and methyl orange alkalinity as follows and express in mg/L as CaCO3,
A*1000
P Alkalinity m mg/L as CaCO3 =
ml sample
B*1000
T Alkalinity m mg/L as CaCO3 =
ml sample
Where,
A= ml of H2SO4 required to raise pH up to 8.3
B= ml of H2SO4 required to raise pH up to 4.5 |
Procedure
Measure a suitable volume of sample in 250 ml conical flask. Add 2-3 drops of phenolphthalein indicator. If the pink colour develops titrate against 0.02 N H2SO4, till the colour disappears, which is the characteristic of pH 8.3.Note down the volume of H2SO4 consumed. Add 2-3 drops of methyl orange and continue titration with H2SO4 till the yellow colour changes to orange, which is the characteristic of pH 4.5. Note down the additional amount of H2SO4 required. In case pink colour does not appear after addition of phenolphthalein, continue with addition of methyl orange. |
Apparatus: Lab glassware - measuring cylinder, pipette, conical flask, etc. |
Reagents
- Standard sulphuric acid (0.02N): Dilute 200 ml of 0.1N H2SO4 with distilled water to 1000ml to obtain standard 0.02N H2SO4.
- Phenolphthalein indicator: Dissolve 0.5g phenolphthalein in 1:1 of 95 % ethanol and distilled water. Add 0.05 N CO2 free NaOH solution drop wise until faint pink colour appears.
- Methyl orange indicator: Dissolve 0.5 g of methyl orange in 1000ml CO2 free distilled water.
|
Do’s and Dont’s
- Ensure that the Erlenmeyer flasks to be used are washed properly and labeled.
- Handle the reagents and acids with extreme care.
|
Results |
Date |
S1 |
S2 |
S3 |
|
Limits (Inland) |
|
|
|
|
|
Permissible
Excessive
500 |
Inference |
|
References |
APHA (1998); Trivedi R. K. and Goel P. K. (1986); Ramachandra T. V. and Ahalya N. (2001) |
TOTAL HARDNESS |
12 |
TOTAL HARDNESS: Water hardness is the traditional measure of the capacity of water to react with soap, hard water requiring a considerable amount of soap to lather.Hardness is predominantly caused by divalent cations such as calcium, magnesium and alkaline earth metals such as iron, manganese, strontium, etc. The total hardness is defined as the sum of calcium and magnesium concentrations, both expressed as CaCO3 in mg/L. Carbonates and bicarbonates of calcium and magnesium cause temporary hardness. Sulphates and chlorides cause permanent hardness.
In alkaline conditions EDTA (Ethylene-diamine tetra acetic acid) and its sodium salts react with cations forming a soluble chelated complex when added to a solution. If a small amount of dye such as Eriochrome black-T is added to aqueous solution containing calcium and magnesium ions at alkaline pH of 10.0 ± 0.1, it forms wine red colour. When EDTA is added as a titrant, all the calcium and magnesium ions in the solution get complexed resulting in a sharp colour change from wine red to blue, marking the end point of the titration. Hardness of water prevents lather formation with soap rendering the water unsuitable for bathing and washing. It forms scales in boilers, making it unsuitable for industrial usage. At higher pH >12, Mg++ ion precipitates with only Ca++ in solution. At this pH, murexide indicator forms a pink color with Ca++ ion. When EDTA is added Ca++ gets complexed resulting in a change from pink to purple indicating end point of the reaction.
METAL + INDICATOR METAL-INDICATOR COMPLEX (WINE RED COLOUR)
METAL-INDICATOR COMPLEX + EDTA METAL-EDTA COMPLEX +
INDICATOR (BLUE COLOUR)
Calculation:
Total hardness (mg/L) = (T) (1000) / V
Where, T = Volume of titrant
V = Volume of sample
Hardness Chart (for drinking water):
Soft |
0 – 60 mg/L |
Medium |
60 –120 mg/L |
Hard |
120 - 180 mg/L |
Very Hard |
> 180 mg/L |
|
Procedure
The complete titration should be completed within 5 minutes after addition of the buffer. Take 25 ml of the sample (dilute it with distilled water if required) and add 1-2 ml of the buffer solution and 1-2 g of EBT indicator. Then titrate against the EDTA, with continuous stirring; until the last reddish tinge disappears. At the end point the solution gives blue colour. |
Apparatus: Lab glassware-burette, pipette, conical flask, beakers etc.
|
Reagents:
- Buffer solution: (a) 16.9 g of ammonium chloride was dissolved in 143ml of concentrated ammonium hydroxide. (b) Dissolve 1.179 g of disodium EDTA and 0.780 g of MgSO4. 7H2O in 50 ml distilled water. Mix both (a) and (b) and dilute to 250ml with distilled water
- Standard EDTA indicator: (0. 01 M) Weigh 3.723 g of EDTA and dissolve in 1000 ml of distilled water and standardize against standard calcium solution.
- EBT Indicator: Mix 0.40 g of Eriochrome Black T with 100 g NaCl (AR) and grind.
|
Do’s and Dont’s
- Ensure that the Erlenmeyer flasks to be used are washed properly and labeled.
- Don’t keep the reagent bottle open.
- Handle the reagents and acids with extreme care.
|
Results |
Date |
S1 |
S2 |
S3 |
|
Limits (Inland) |
|
|
|
|
|
Permissible
150
Excessive
500 |
Inference |
|
References |
APHA (1998); Trivedi R. K. and Goel P. K. (1986); Ramachandra T. V. and Ahalya N. (2001) |
CALCIUM HARDNESS |
13 |
CALCIUM HARDNESS: The presence of calcium (fifth most abundant) in water results from passage through or over deposits of limestone, dolomite, gypsum and such other calcium bearing rocks. Calcium is present in all waters as Ca2+ and is readily dissolved from rocks rich in calcium minerals, particularly as carbonates and sulphates. Calcium carbonate solubility is controlled by pH and dissolved CO2. The salts of calcium, together with those of magnesium are responsible for hardness of water. Calcium is an important element for all organisms and is incorporated in to the shells of many aquatic invertebrates, as well as the bones of vertebrates. Calcium concentrations in natural waters are typically <15 mg/l. For water associated with carbonate rich rocks, concentrations may reach 30-100 mg/l. Salt waters have concentrations of several hundred milligrams per litre or more.
When EDTA (Ethylene-diamine tetra acetic acid) is added to the water containing calcium and magnesium, it combines first with calcium. Calcium can be determined directly with EDTA when pH is made sufficiently high such that the magnesium is largely precipitated as hydroxyl compound (by adding NaOH and iso-propyl alcohol). When murexide indicator is added to the solution containing calcium, all the calcium gets complexed by the EDTA at pH 12-13. The end point is indicated from a colour change from pink to purple.
Calculation
A*B*1000
Calcium hardness as CaCO3 = * Dilution factor (If diluted)
ml of sample taken
Where, A = ml titrant for sample and B = mg Caco3 equivalent to 1.0 ml EDTA titrant. |
Procedure
A known volume (25ml) of the sample is pipetted into a clean conical flask, to which 1ml of sodium hydroxide is added. A pinch of murexide indicator is added to this mixture and titrated against EDTA until the pink color turns purple. |
Apparatus: Burettes, pipette, conical flask, beakers and droppers
|
Reagents:
- Sodium hydroxide (8%):8g of sodium hydroxide is dissolved in 100ml of distilled water.
- Murexide indicator (ammonium purpurate):0.2 g of murexide is ground well with 100g of sodium chloride.
- Standard EDTA titrant, 0.01M:3.723 g of EDTA (disodium salt) is dissolved in distilled water and made up to 100ml with the same.
|
Do’s and Dont’s
- Ensure that the Erlenmeyer flasks to be used are washed properly and labeled.
- Don’t keep the reagent bottle open.
- Handle the reagents and acids with extreme care.
|
Results |
Date |
S1 |
S2 |
S3 |
|
Limits (Inland) |
|
|
|
|
|
Permissible
75
Excessive
200
*Calcium (as Ca) mg/l |
Inference |
|
References |
APHA (1998); Trivedi R. K. and Goel P. K. (1986); Ramachandra T. V. and Ahalya N. (2001) |
MAGNESIUM HARDNESS |
14 |
MAGNESIUM HARDNESS: Magnesium is a relatively abundant element in the earth's crust, ranking eighth in abundance among the elements. It is found in all natural waters and its source lies in rocks, generally present in lower concentration than calcium. It is also an important element contributing to hardness and a necessary constituent of chlorophyll. Its concentration greater than 125 mg/L can influence cathartic and diuretic actions.
Magnesium hardness can be calculated from the determined total hardness and calcium hardness.
Magnesium = (T - C) x 0.243 (as mg/L)
where, T = Total hardness mg\L (as CaCO3)
C = Calcium hardness mg\L (as CaCO3)
High concentration of magnesium proves to be diuretic and laxative, and reduces the utility of water for domestic use while a concentration above 500 mg/L imparts an unpleasant taste to water and renders it unfit for drinking. Chemical softening, reverse osmosis and electro dialysis or ion exchange reduces the magnesium hardness to acceptable levels. |
Results |
Date |
S1 |
S2 |
S3 |
|
Limits (Inland) |
|
|
|
|
|
Permissible
50
Excessive
150
*Magnesium (as Mg) mg/l |
Inference |
|
References |
APHA (1998); Trivedi R. K. and Goel P. K. (1986); Ramachandra T. V. and Ahalya N. (2001) |
CHLORIDES |
15 |
CHLORIDES: Chloride (Cl-) enters surface waters by the atmospheric deposition of oceanic aerosols with the weathering of some sedimentary rocks (mostly rock salt deposits), industrial effluents and agricultural run-off. High concentrations of chloride can make waters unpalatable and therefore unfit for drinking or livestock watering. Chloride is an abundant anion found in the wastewaters and is a good indicator of pollution sources. Chloride gives water a salty taste detectable at a level of 250 ppm with sodium as cation, but with Ca and Mg cations, the salty taste is not detectable until the chloride concentration reaches up to 1000 ppm. High chloride content has a deleterious effect on metallic pipes and structures, as well as agricultural plants. They are calculated by Argentometric method.
In alkaline or neutral solution, potassium chromate indicates the endpoint of the silver nitrate titration of chlorides. Silver chloride is quantitatively precipitated before the red silver chromate is formed.
Ag++ Cl- AgCl (White precipitate)
2Ag++CrO-4 Ag2CrO4 (Red precipitate)
Calculation
(A-B)*N*35.45*1000
Chlorides mg/L =
ml sample
Where, A = ml Ag NO3 required for sample.
B = ml Ag NO3 required for blank. |
Procedure
A known volume of filtered sample (50ml) is taken in a conical flask, to which about 0.5ml of potassium chromate indicator is added and titrated against standard silver nitrate till silver chromate (Ag2CrO4) starts precipitating. |
Apparatus: Lab glassware, pipette.
|
Reagents:
- Potassium chromate indicator solution:50g of potassium chromate is dissolved in minimum amount of distilled water and silver nitrate is added drop wise till a red precipitate is formed. The mixture is allowed to stand for about 12 hours and diluted to 1000ml with distilled water.
- Silver nitrate solution (0.014N):2.395g of silver nitrate is dissolved in distilled water and made up to 1000ml.
|
Do’s and Dont’s
- Ensure that the Erlenmeyer flasks to be used are washed properly and labeled.
- Don’t keep the reagent bottle open.
- Handle the reagents and acids with extreme care.
|
Results |
Date |
S1 |
S2 |
S3 |
|
Limits (Inland) |
|
|
|
|
|
Permissible
250
Excessive
600 |
Inference |
|
References |
APHA (1998); Trivedi R. K. and Goel P. K. (1986); Ramachandra T. V. and Ahalya N. (2001) |
POTASSIUM |
16 |
POTASSIUM: Potassium ranks seventh among the elements in order of abundance, behaves similar to sodium and remains low. Though found in small quantities (<20mg/L), it plays a vital role in the metabolism of fresh water environment.
Trace amounts of potassium can be determined by direct reading of flame photometer at a specific wavelength of 766.5nm by spraying the sample into the flame. The desired spectral lines are then isolated by the use of interference filters or suitable slit arrangements. The intensity of light is measured by the phototube.
Working principle of Flame photometer: The emission of characteristic radiations by alkali and alkaline earth metals and the correlation of the emission intensity with the concentration of the element form the basis of flame photometry. The principle of the flame photometer depends on the "Emission Spectroscopy" in which the electrons of the metals after absorbing energy get excited from ground state to higher energy level and return back to the ground state with emission of light. The sample under test is introduced into flame in solution by means of atomizer. The radiation from the flame enters a dispersing device and isolates it (radiation) from the flame to the desired region of the spectrum. The photo tube measures the intensity of isolated radiation, which is proportional to the concentration of the element present in the sample.
Calculation:
Potassium (K) or Sodium (Na), mg/L
= (mg/L of K/Na in diluted aliquot) x dilution factor |
Procedure
The filter of the flame photometer is set at 766.5nm (marked for Potassium, K) and the flame is adjusted for blue colour. The scale is set to zero and maximum using the highest standard value. A standard curve of different concentration is prepared by feeding the standard solutions. The sample is filtered through the filter paper and fed into the flame photometer. The concentration is found from the standard curve or as direct reading. |
Apparatus: Flame photometer, lab glassware and Whattman filter paper. |
Do’s and Dont’s
- Ensure that the Erlenmeyer flasks to be used are washed properly and labeled.
- Don’t keep the reagent bottle open.
- Handle the reagents and acids with extreme care.
|
Results |
Date |
S1 |
S2 |
S3 |
|
Limits (Inland) |
|
|
|
|
|
Permissible
Excessive |
Inference |
|
References |
APHA (1998); Trivedi R. K. and Goel P. K. (1986); Ramachandra T. V. and Ahalya N. (2001) |
SODIUM |
17 |
SODIUM: Sodium is one of the most abundant elements and is a common constituent of natural waters. The sodium concentration of water is of concern primarily when considering their solubility for agricultural uses or boiler feed water. The concentration ranges from very low in the surface waters and relatively high in deep ground waters and highest in the marine waters. It is calculated by flame photometric method. |
Procedure
The filter of the flame photometer is set to 589nm (marked for Sodium, Na). By feeding distilled water the scale is set to zero and maximum using the standard of highest value. A standard curve between concentration and emission is prepared by feeding the standard solutions. The sample is filtered through filter paper and fed into the flame photometer and the concentration is found from graph or by direct readings. |
Apparatus: Flame photometer, lab glassware and Whattman filter paper. |
Do’s and Dont’s
- Ensure that the Erlenmeyer flasks to be used are washed properly and labeled.
- Don’t keep the reagent bottle open.
- Handle the reagents and acids and gas of flame photometer with extreme care.
|
Results |
Date |
S1 |
S2 |
S3 |
|
Limits (Inland) |
|
|
|
|
|
Permissible
Excessive |
Inference |
|
References |
APHA (1998); Trivedi R. K. and Goel P. K. (1986); Ramachandra T. V. and Ahalya N. (2001) |
NITRATES |
18 |
NITRATES: Nitrate ion (NO3¯) is the common form of combined nitrogen found in natural waters. It may be bio chemically reduced to nitrite (NO2¯) by denitrification processes, usually under anaerobic conditions. The nitrite ion is rapidly oxidized to nitrate. Natural sources of nitrate to surface water include igneous rocks, domestic sewage, land drainage and plant growth and decay. The natural concentration of nitrate is 0.1mg/L; it may be enhanced by sewage, industrial effluents and nitrate fertilizers.
Ammonia and other nitrogenous material in natural waters tend to be oxidized by aerobic bacteria first to nitrite and then nitrates. So all organic compounds containing nitrogen are potential source of nitrates. It is first oxygenated to nitrite (NO2¯) and is rapidly oxidized to nitrate (NO3¯) so normally the amount of nitrite is very low. High concentrations (>1-2 mg/L) of nitrate or nitrite in surface or ground water generally indicate agricultural contamination from fertilizers and manure.
NITRIFICATION
(O) (R)
 Ammonia Nitrite Nitrates
ADB FAB
ADB = Aerobic Denitrifying Bacteria. Ex: Nitrosomes
FAB = Facultative Anaerobic Denitrifying Bacteria. Eg: Pseudomonas
Estimation of nitrates by electrode screening method
The NO3¯ ions electrode is a selective sensor that develops a potential across a thin, porous, inert membrane that holds in a place a water-immiscible liquid ion exchanger. The electrode responds only to NO3¯ ion activity between about 10¯5 and 10¯1 M (0.14 to 1400 mg NO3¯ -N/L). The lower limit of detection is determined by the small but finite solubility of the liquid ion exchanger. |
Procedure
A known volume (50ml) of the sample is pipetted into a porcelain dish and evaporated to dryness on a hot water bath. 2ml of phenol disulphonic acid is added to dissolve the residue by constant stirring with a glass rod. Concentrated solution of sodium hydroxide or conc. ammonium hydroxide and distilled water is added with stirring to make it alkaline. This is filtered into a Nessler's tube and made up to 50ml with distilled water. The absorbance is read at 410nm using a spectrophotometer after the development of colour. The standard graph is plotted by taking concentration along X-axis and the spectrophotometric readings (absorbance) along Y-axis. The value of nitrate is found by comparing absorbance of sample with the standard curve and expressed in mg/L. |
Apparatus: Porcelain dishes, pipettes, standard flasks, beakers, spectrophotometer, cuvettes, measuring jar and hot water bath.
|
Reagents:
Phenol disulphonic acid:25 g of phenol is dissolved in 150 ml of concentrated sulphuric acid, to which 85ml of sulphuric acid is further added and heated for about 90 min on a water bath and stored in dark bottles upon cooling.
Sodium hydroxide:About 50g of sodium hydroxide is dissolved in 150-200 ml of water and cooled.
Conc. Ammonium hydroxide (Liquid ammonia)
Nitrate solution:
- Stock nitrate solution:721.8 mg (0.722g) of AR potassium nitrate is dissolved in distilled water and made up to 100ml for stock solution.
- Standard nitrate solution:Standard nitrate solution is prepared by evaporating 50ml of the stock solution to dryness in the water bath. The obtained residue is dissolved in 2ml of phenol disulfonic acid and diluted to 500ml, to give 1ml = 10 mg. The solution of various strengths ranging from 0.0 (blank) to 1.0 mg/L at the intervals of 0.2 mg/L is prepared by diluting stock solution with distilled water.
|
Do’s and Dont’s
- Ensure that the Erlenmeyer flasks to be used are washed properly and labeled.
- Don’t keep the reagent bottle open.
- Handle the reagents and acids with extreme care.
|
Results |
Date |
S1 |
S2 |
S3 |
|
Limits (Inland) |
|
|
|
|
|
Permissible
45
Excessive |
Inference |
|
References |
APHA (1998); Trivedi R. K. and Goel P. K. (1986); Ramachandra T. V. and Ahalya N. (2001) |
PHOSPHATES |
19 |
PHOSPHATES: Phosphates are an essential nutrient for living organisms and exist in water bodies on both forms as dissolved and particulate species. Phosphorus concentration is limiting factor for algae growth. Artificial increase in phosphorous concentration in aquatic system results in eutrophication. In natural water and wastewater phosphorous occurs as orthophosphates, polyphosphates and organically bound phosphates.
Orthophosphates applied to agricultural land as a fertilizer, finds its way to the surface water by runoff. The condensed phosphate like pyrophosphates and polyphosphates comes to environment from the heavy-duty detergents (Sodium tri polyphosphate Na5P3O10). Biological processes are the only source for organic phosphates, such as weathering of rock and decomposition of organic matter.
Estimation of phosphorous by stannous chloride Method
In the acidic condition, orthophosphates reacts with ammonium molybdate to form molybdophosphoric acid. It is further reduced to intensely coloured molybdenum blue colour by adding the reducing agent stannous chloride. The intensity of the coloured complex is measured at 690nm, which is directly proportional to the concentration of phosphates present in the sample.
PO4+12(NH4)2MoO4+24H+ (NH4)PO4.12MoO3+21NH4++12H2O
(NH4)3PO4.12 MoO3+Sn2+ Molybdenum blue +Sn4+
Calculation
Mg/L of phosphates = Test absorbance * Slope * Dilution factor
Sum of concentration of Standards
Where, Slope =
Sum of absorbance of Standards
ml sample + ml distilled water
Dilution ratio (D) =
ml sample |
Procedure
Take the appropriate aliquot of sample (as per the expected concentration of phosphates) in the Nessler tube. Add 2ml of ammonium molybdate and mix well. Add 0.5 ml of stannous chloride and make up to the mark with distilled water. Prepare the blank using the distilled water instead of sample. Measure the intensity of light path at 690 nm.
|
Apparatus: Spectrophotometer, lab glassware, hot plate and Nessler's tube. |
Reagents
- Standard phosphate solution: Dissolve 219.5 mg of anhydrous KH2PO4 in 1000ml of water (1 ml = 0.5 mg PO4).
- Ammonium molybdate solution:
a) Dissolve 25 g ammonium molybdate in 175 ml distilled water.
b) Add cautiously 280 ml concentrated sulphuric acid to 400ml water and cool.
Mix two solutions (a) and (b) and make up to 1000ml.
- Stannous Chloride: Dissolve 2.5 g SnCl2. 2 H2O in the 100ml glycerol. Heat in water bath to ensure complete dissolution. Prepare stannous chloride freshly.
|
Do’s and Dont’s
- Ensure that the Erlenmeyer flasks to be used are washed properly and labeled.
- Don’t keep the reagent bottle open.
- Handle the reagents and acids with extreme care.
|
Results |
Date |
S1 |
S2 |
S3 |
|
Limits (Inland) |
|
|
|
|
|
Permissible
2
Excessive
5 |
Inference |
|
References |
APHA (1998); Trivedi R. K. and Goel P. K. (1986); Ramachandra T. V. and Ahalya N. (2001) |
Table: Surface Water Quality Standards (as per IS: 2296).
Class A – Drinking water without conventional treatment but after disinfection. Class B –Water for outdoor bathing. Class C – Drinking water with conventional treatment followed by disinfection. Class D – Water for fish culture and wild life propagation. Class E – Water for irrigation, industrial cooling and controlled waste disposal. (Unobj = Unobjectionable).
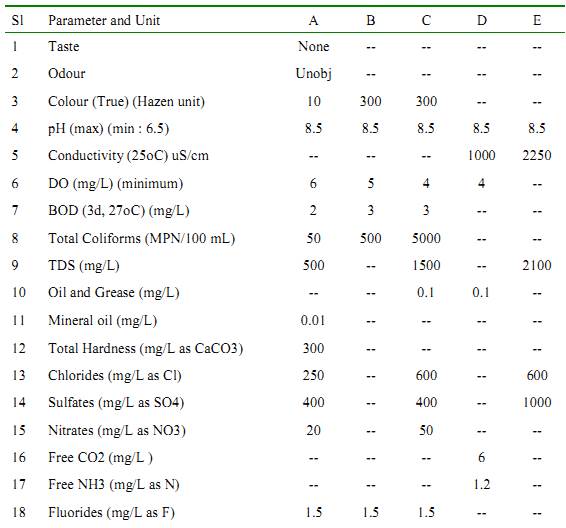
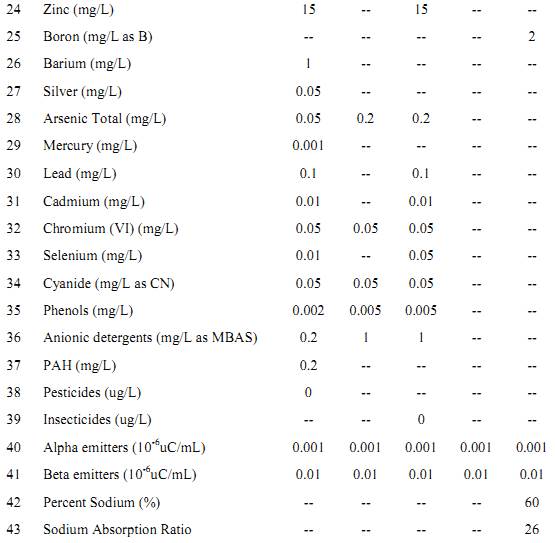
BIOLOGICAL PARAMETERS |
PLANKTON ANALYSIS: The physical and chemical characteristics of water affect the abundance, species composition, stability and productivity of the indigenous populations of aquatic organisms. The biological methods used for assessing water quality includes collection, counting and identification of aquatic organisms; biomass measurements; measurements of metabolic activity rates; toxicity tests; bioaccumulation; biomagnifications of pollutants; and processing and interpretation of biological data. The work involving plankton analysis would help in:
- Explaining the cause of colour and turbidity and the presence of objectionable odour, tastes and visible particles in waters.
- The interpretation of chemical analyses.
- Identifying the nature, extent and biological effects of pollution.
- Providing data on the status of an aquatic system on a regular basis.
|
Plankton:A microscopic community of plants (phytoplankton) and animals (zooplankton), found usually free floating, swimming with little or no resistance to water currents, suspended in water, non-motile or insufficiently motile to overcome transport by currents, are called "Plankton".
Phytoplankton (microscopic algae) usually occurs as unicellular, colonial or filamentous forms and is mostly photosynthetic and is grazed upon by the zooplankton (microscopic protozoans, rotifers, cladocerans and copepods) and other organisms occurring in the same environment.
The structure of photosynthetic populations in the aquatic ecosystems is dynamic and constantly changing in species composition and biomass distribution. An understanding of the community structure is dependent on the ability to understand the temporal distribution of the different species. Changes in species composition and biomass may affect photosynthetic rates, assimilation efficiencies, rates of nutrient utilization, grazing, etc.
Plankton, particularly phytoplankton, has long been used as indicators of water quality. Because of their short life spans, planktons respond quickly to environmental changes. They flourish both in highly eutrophic waters while a few others are very sensitive to organic and/or chemical wastes. Some species have also been associated with noxious blooms causing toxic conditions apart from the tastes and odour problems. |
Plankton net:The plankton net is a field-equipment used to trap plankton. It has a polyethylene filter of a defined mesh size and a graduated measuring jar attached to the other end. A handle holds the net. The mesh size of the net determines the size range of the plankton trapped. The mesh number 30 of size 60 mm was used for collecting samples. |
Sampling Procedure:The manner in which sampling is done should conform to the objectives of the study. The “surface samples” (samples collected from the surface) are collected as close to the water surface as possible, mostly towards the center of the lake at regular monthly intervals. A known volume of the sample, 5L to 50 L is filtered and planktons are filtered and preserved for further analysis. |
Labels:The sample label has the date, time of sampling, study area-lake name and the volume measured and pasted on the containers of 50 ml capacity. |
Preservation:The samples are collected into 100 ml polyethylene vials and are preserved by adding suitable amounts of 1ml chloroform to act as the narcotizing agent and 2 ml of 2% formalin for preservation and analyses. |
Concentration technique: The plankton nets are used to collect samples for the qualitative and quantitative estimation of the plankton, by filtering a known volume of water (5-50 liters) through the net depending on the plankton density of the tanks. |
Qualitative and quantitative evaluation of plankton:Detailed analyses of phytoplankton populations are done by estimating the numbers in each species. The phytoplankton consisting of individual cells, filaments and colonies are counted as individual cells. When colonies of species are counted, the average number of cells per colony is counted, and in filamentous algae, the average length of the filament has to be determined. |
Sedimentation and enumeration by microscope:Preserved samples in bottles are mixed uniformly by gentle inversion and then exactly 1ml of the sample is pipetted out into the S-C cell for analysis. |
Microscope
Compound microscope: A monocular compound microscope is used in the counting of plankton with different eyepieces such as 4X, 10X and 40X. The microscope is calibrated using plankton-counting squares. |
Counting
Counting cell- Sedgwick-Rafter (S-R) cell: The Sedgwick-Rafter cell is a devise used for plankton counting and is about 50mm long by 20mm wide and 1mm deep. The cell is covered by a relatively thick cover slip and is calibrated to contain exactly 1.0 ml.
Method
Filling the cell:The cover slip is placed diagonally across the S-R cell and filled with the sample carefully without air bubbles with a large bore pipette. The sample is allowed to settle for about 5 minutes before the actual counting begins. |
Note: Since the configuration of the S-R cell does not allow the use of high power microscope objectives, the identification of organisms smaller than 10 – 15 mm is difficult or impossible, limiting the usage to only larger forms of relatively dense populations.
Strip counting: A "strip" is the length of the cell that constitutes a volume approximately 50 mm long, 1-mm deep accounting to the volume of 25mm3 or 1/40 (2.5%) of the total cell volume. By moving the mechanical stage from left to right, the organisms can be examined in a systematic manner. By knowing the surface area of the portion counted in relation to that of the total, a factor is determined to expand the average counts of the plankton to the total area of the counting surface. This total area represents the number of organisms present per given volume of the sample. This volume expanded to an appropriate factor yields the organisms per litre of water for the lake.
The total number of planktons in the S-R cell is obtained by multiplying actual count in the strip by the number (enumeration factor) representing the portion of the S-R cell counted. The number of the strips counted is a function of the precision desired and the number of units (cells, colonies) for the strips measured. In this study, 500 cells were counted for estimation.
The plankton count in the S-R cell is got from the following,
C X 1000 mm
Number/ml = -------------------------
L X D X W X S
Where
C = Number of organisms counted
L = Length of each strip (S-R cell length) mm
D = Depth of a strip (S-R cell depth) mm
W = Width of a strip in mm
S = Number of strips counted
V1 = (50)(1)(W)
= mm3
The plankton counts per strip are then determined by multiplying the actual count by the factor representing the counted portion of the whole S-R cell volume.
Number/ml = (C) (1000 mm3) / (L) (D) (W) (S)
Where
C = Number of organisms counted.
L = Length of each strip in mm (of S-R cell)
D = Depth of the strip in mm (S-R cell)
W = Width of the strip in mm (Whipple grid image width)
S = Number of strips counted.
Phytoplankton Counting Units: Some plankton are unicellular while others are multicellular (colonial), posing a problem for enumeration. For analysis, a colony of plankton is accounted as a single count. The large forms that cross two or more boundaries of the grid are counted separately at lower magnification and their number included in the total count. |
COMMONLY FOUND ALGAL GROUPS IN LAKES |
Bacillariophytes: They occur in fresh water, salt water, and terrestrial ecosystems. They are single-celled algae with shells constructed of two overlapping valves composed of pectin and impregnated with silica. Amorphous silica is the form of silicon in diatom cell walls. The diatoms are single-celled or unicellular organisms, but some can form colonies and filaments. They may occur as plankton or periphyton, with most brownish-green films on substrates such as rocks or aquatic plants being composed of attached diatoms. The Bacillariophytes comprises two main types: centric and pennate. Centric diatoms are radially symmetrical and pennate diatoms that are bilaterally symmetrical. Many diatoms have conspicuous oil droplets within the cell, which is the photosynthetic food reserve, chrysolaminarin. The diatoms contain the pigments chlorophyll a and b, alpha and beta carotene, and several xanthophylls.
Example: Nitzschia sp., Cymbella sp., Melosira sp., Navicula sp., Gomphonema sp., Cyclotella sp., Pinnularia sp., Gyrosigma sp., etc. |
Euglenoids: Typically green and unicellular, euglenoid flagellates occur in most freshwater habitats: puddles, ditches, ponds, streams, lakes, and rivers, particularly waters contaminated by animal pollution or decaying organic matter. Most are photosynthetic, but many, lacking chloroplasts are heterotrophs. Most do not reproduce sexually. Euglenoids lack a cellulose cell wall; instead, they have a proteinaceous pellicle just inside the plasmalemma. Euglenoids are characterized by chlorophylls a, b, beta carotene, and xanthophylls, one membrane of chloroplast endoplasmic reticulum, a mesokaryotic nucleus, two emergent flagella with fibrillar hairs in one row and paramylon or chrysolaminarin as the storage product in the cytoplasm. They have eyespot (stigma) which is a collection of orange-red lipid droplets. If placed in the dark over the course of several divisions, the chloroplasts of Euglena gracilis will become colorless. When returned to the light, the plastid structure is reformed and the green color returns. All euglenoid species with an eyespot and flagellar swelling exhibit phototaxis, usually swimming away from bright light (negative phototaxis) and away from darkness toward subdued light (positive photoaxis) to accumulate in a region of low light intensity.
Example: Euglena sp., Phacus sp., Trachelomonas sp., etc. |
Blue-Green Algae (Cyanobacteria): The cyanophytes are the only prokaryotic algae and lack membrane bound organelles. Cyanobacteria are aerobic phototrophic organisms that can perform photosynthesis as they possess chlorophyll a and photosystem II. They are ubiquitous in nature and are found in every type of environment including terrestrial, freshwater, and marine habitats. Blue green algae are very common in waters of a great range of salinity and temperature, and they occur in and on the soil and also on rocks and in their fissures. However, the external structure can range from unicellular or colonial to branched or unbranched and filamentous. The cyanobacterial cells possess a number of cell inclusion bodies like include phycobilisomes, carboxysomes, glycogen granules, polyphosphate granules, poly-β –hydroxybutyrate granules, cyanophycin granules and gas vesicles. The cyanophytes possess no flagellated or cilliated cells at any stage of their lifecycle, although, simple movements such as bending and swaying are made possible by internal pressure changes exerted on the cell wall. They are heavily pigmented with chlorophyll a, beta carotene, and several xanthophylls. The presence of several phycobiliproteins gives the cyanophyta their unique blue-green coloration. Food is stored in the form of glycogen. Example: Microcystis sp., Merismopedia sp., Phormidium sp., Anabaena sp., etc. |
Green Algae (Chlorophytes): Chlorophytes are a diverse group and are common in fresh water, salt water, and soil. The chlorophyta are primarily freshwater; only about 10% of the algae are marine, whereas 90% are freshwater. Green algae have unicellular or multicellular thalli. Some are flagellates, and others produce reproductive cells, the majority of which are biflagellate. Chlorophyte reproduction varies greatly, from asexual division to isogamy and heterogamy to oogamy. Cell walls are constructed of cellulose and pectin. The food storage product is true starch, the same as plants. Green algae possess true chloroplasts, which contain the same pigments found in higher plants: chlorophyll a and b, alpha and beta carotene, and many xanthophylls.
Example: Chlorella sp., Ankistrodesmus sp., Chlamydomonas sp., Pandorina sp., Eudorina sp., Chlorogonium sp., Actinastrum sp., Pediastrum sp., Scenedesmus sp., etc. |
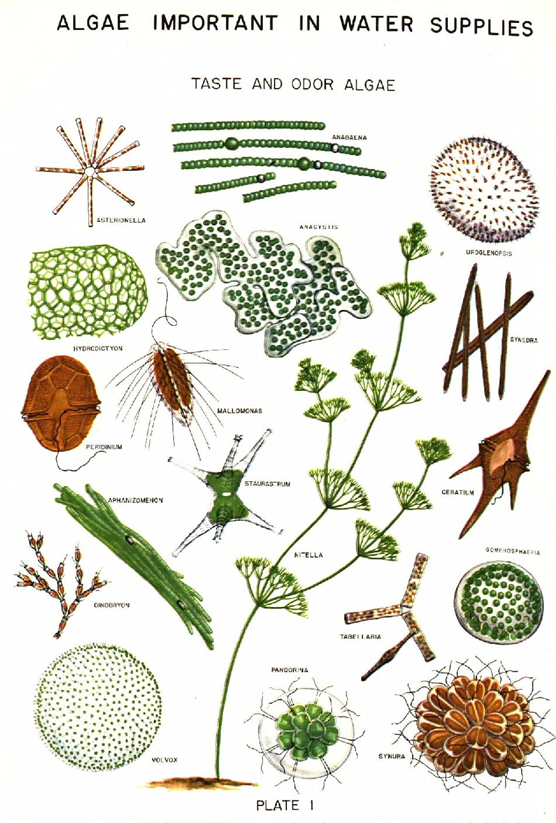
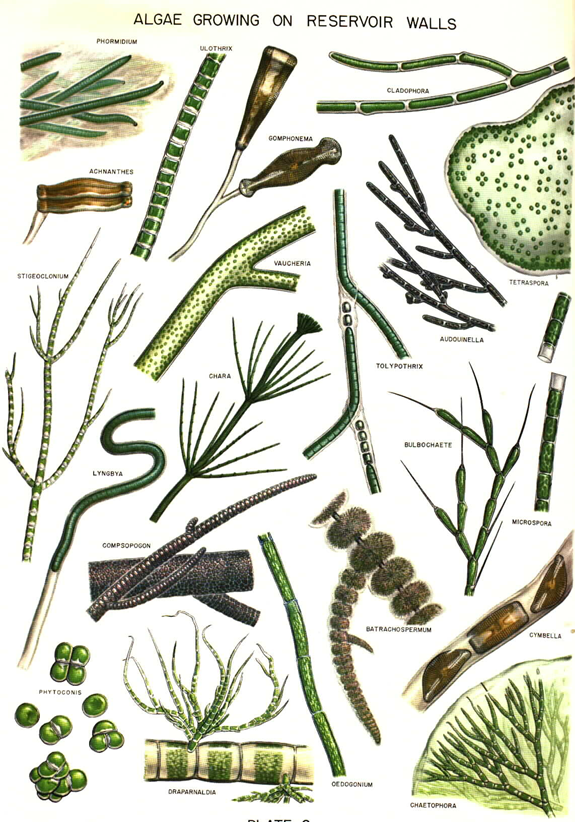
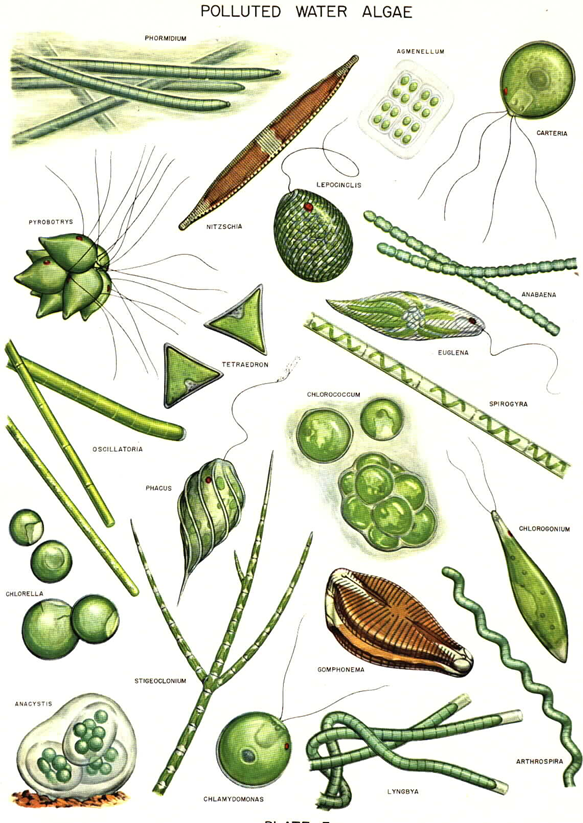
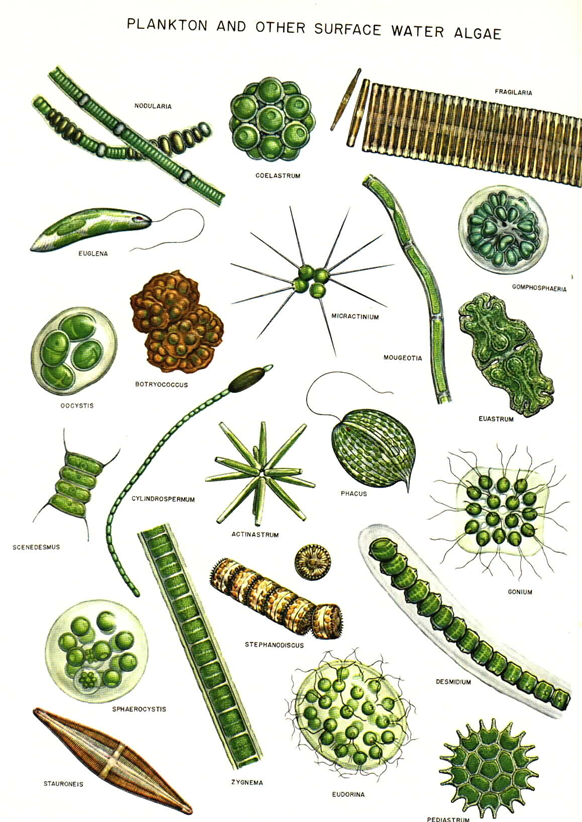
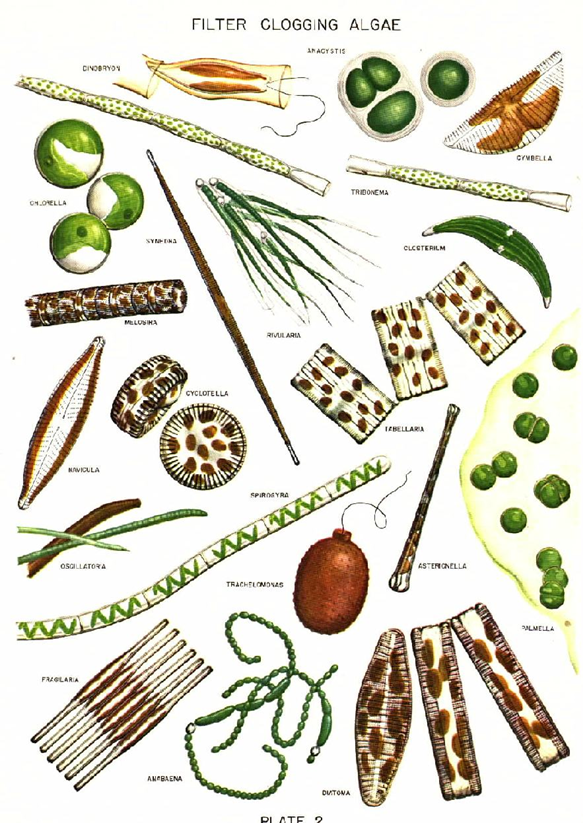
Macrophytes (Aquatic plants) commonly found in Bangalore
Name:Eichhornia crassipes
Common Name: Water hyacinth
Description: Water hyacinth is a free-floating perennial aquatic plant with broad, thick, glossy, ovate leaves; leaves are 30-40 cm long with spongy petiole. Roots are fibrous and featherlike.
Flowering: March-July
Habitat: Water hyacinth grows in still or slow-flowing fresh water in tropical and temperate climates. Optimum growth occurs at temperatures of between 28°C and 30°C, and requires abundant nitrogen, phosphorus and potassium.
Impact: Its wide spread occurrence in the fresh water lakes and riverbeds is harmful to fishing (depleting DO), rowing, and depleting water content from the water bodies and interfering in water utilization and other activities. Water hyacinth by its abundance of leaves, dense vegetation and innumerable rootlets in tertiary manner obstruct water flow in irrigation channels and displaces many aquatic grasses, which were useful as fodder for cattle, and suppresses the phytoplankton growth. Water hyacinth provides suitable breeding places for mosquitoes and other disease-carrying insects by stagnating the water in ditches and shallow areas.
Uses: Phytoremediation, waste water treatment |
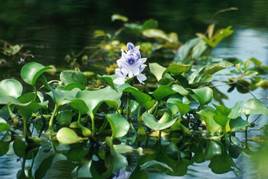
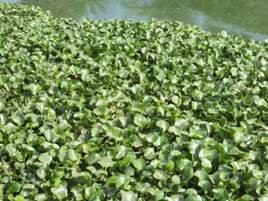
|
Name: Alternanthera philoxeroides
Common name: Alligator weed
Habitat: grow in a variety of habitats, including dry land but usually found in water.
Stems are pinkish, long, branched, and hollow. Fleshy, succulent stems can grow horizontally and float on the surface of the water, forming rafts, or form matted clumps which grow onto banks
Leaves are simple, elliptic, and have smooth margins. They are opposite in pairs or whorls, with a distinctive midrib, and range in size from 5-10 cm.
Flowers: whitish, papery ball-shaped flowers that grow on stalks.
Fibrous roots arising at the stem nodes may hang free in water or penetrate into the sediment/soil.
Flowering: December-April
Impact: Alligator weed disrupts the aquatic environment by blanketing the surface and impeding the penetration of light. Such blanketing can also prevent gaseous exchange (sometimes leading to anaerobic conditions) which adversely affects aquatic flora and fauna. It also competes with and displaces native flora along river and in wetlands |
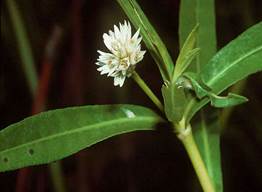
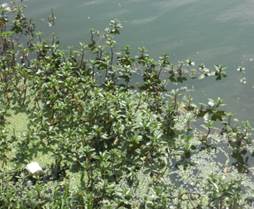
|
Name: Typha
Common name: Cattail
Description: It is a common perennial marsh, aquatic or wetland plant in temperate, tropical, and subtropical climates. Plants are rhizomatous monoecious herb, grow upto 1.5-3m high, leaves radical, sheath white. Flowering stem length is typically equal to or somewhat longer than leaf length. Numerous tiny, dense, flowers occur in a terminal spike that is 0.7 to 2 inches, Male flowers make up the upper, narrower half of the spike and female flowers the lower, slightly wider half
Flowering: June- August
Habitat: It grows in shallow water of lakes, rivers, ponds, marshes, and ditches.
Significance: Phytoremediation, wastewater treatment, used as medicine and fodder.
|
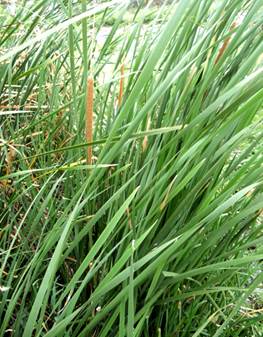
|
Name: Lemna
Common name:Common duckweed
Lemna minor: free-floating aquatic plants, with one, two or three leaves each with a single root hanging in the water; as more leaves grow, the plants divide and become separate individuals. The root is 1-2 cm long. The leaves are oval, 1-8 mm long and 0.6-5 mm broad, light green, with three (rarely five) veins, and small air spaces to assist flotation. It propagates mainly by division, and flowers rarely produced.
Habitat: Grows in water with high nutrient levels and a pH of between 5 and 9, optimally between 6.5 and 7.5, and temperatures between 6 and 33 °C.
Significance: Important food resource for fish and birds(ducks)
|
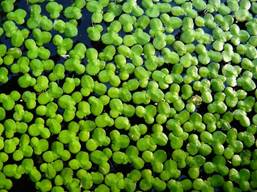
Lemna minor
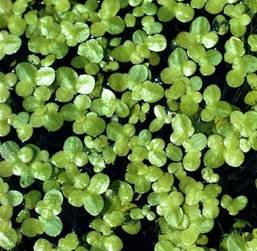
Lemna gibba
|
Name:Pistia stratiotes
Common name: water cabbage, water lettuce
Description: Aquatic free-floating, odorous monoecious herb with thick, soft leaves that form a rosette. Roots hanging submersed beneath floating leaves. Leaves can be up to 14 cm long and have no stem. They are light green, with parallel veins, wavy margins and are covered in short hairs which form basket-like structures which trap air bubbles, increasing the plant's buoyancy.
Habitat: waters with high nutrient content, particularly those that have been contaminated with human loading of sewage or fertilizers
Impact: major weed of lakes, dams, ponds, irrigation channels and slow-moving waterways in tropical, subtropical and warmer temperate regions. It can completely cover water bodies(lowering DO), disrupting all life on the water. |
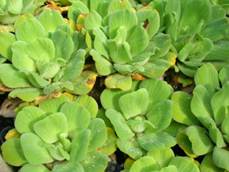
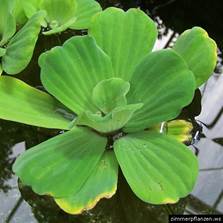 |
|