Introduction
Soil is one of the major abiotic factors which support life on our planet Earth. Soils are natural three dimensional bodies used for many purposes; the most important purpose is for food and fiber (USDA Soil Conservation Service). Soils are natural bodies in which the plant grows. It supports crops, grasslands and forests which will provide food, fiber, wood, fuel and building materials. Great civilizations have almost invariably had good soils as one of their chief natural energy resource. Soil mismanagement was associated with the downfall of some of the same civilization that good soil had helped to build. Even today there are many who didn’t recognize the long term significance of soils. Soil is an important natural resource. The discovery of agriculture leads to the excessive use of soil by man. With the exception of light, soil can provide all the five elements, like mechanical support, heat, air, water and nutrients for the growth of plants (Brady, 1984). Not only it serves to promote and sustain life in its many forms, but it also acts as a living filter for the waste generated by humans and animals.
SOIL AS ECOSYSTEM COMPONENT
The general concept and the definition of soil have been subjected to many alterations (Wilde, 1946). According to agronomists “soils are a mixture of sand, clay, lime and humus”. Geologists regard soil as “products of weathered minerals and a composition of dead plant and animal remaining (Fallow, 1862) and biologists consider it as “a lithosphere penetrated by the biosphere” (Stebutt, 1930). In general soil is “a portion of the earth surface which serves as a medium for the sustenance of biosphere; it consists of mineral and organic matter, permeated by varying amounts of water and air, and inhabited by organisms; it exhibits peculiar characteristics impressed by the physical and chemical action of the tree roots and forest debris (Wilde, 1946). Soil is a vital component of the ecosystem and many activities in the ecosystem like nutrient cycles takes place through the soil. Soils play a crucial role in biogeochemical cycles as spatially distributed sources and sinks of nutrients. The early observations of the relationship that exist between the soil and vegetation were made by the primitive forest dwellers, hunters and medicine men.
With the advance of research in soil science two basic concepts of soil have evolved. One considers that soil is biochemical weathered product and the other considers that soil is entirely for the growth of plants. This has contributed to two approaches used in soil study; that is pedlogy and edaphology (Brady, 1984). Pedlogy mainly deals with the origin, classification, and the description of the soil while edaphology deals with the relation of plant and soil. Plants play a vital role in the formation and the structure of the soil. Vegetation affects the soil structure through different mechanisms like root penetration, water extraction, and anchorage (Angers, 1998)
SOIL FORMATION AND SOIL PROFILE
Soil formation takes place in the earth due to two distinct forces, one is the destructive force and the other is the synthetic force. Microbial decay and the weathering of the parent material are destructive processes, which result in the formation of a new mineral like clay from the parent material, which is a synthetic process. Examination of the vertical section of soil gives an idea of underlying forces in soil formation. The vertical section of the soil is called the profile and the individual layer is called as horizon (Figure 1). Each horizon has its own peculiarities. The upper layer of soil profile has large amount of organic matter. This is mainly due to decaying organic matter. Variation in color of each horizon is evident as the upper layer has dark color compared to the other underlying layers. The underlying layers which form the subsoil have low organic matter.
The uppermost horizon in a typical soil profile is called the O horizon. This horizon is composed mostly of mineral soils. This horizon contains more than 30% of organic matter present in the soil profile. Below the O horizon is the A horizon which is called the fertile top soil. Below the A horizon is the E horizon .The main feature of this horizon is the loss of clay, iron and the aluminum. Mineral horizon with evidence of pedogenesis or illuviation is called the B horizon. Below the B horizon is the un-weathered geologic material commonly called as C horizon. Below the C horizon is the hard bed rock commonly called R horizon (Miller & Donahue, 1990). The quality of the soil varies from horizon to horizon.
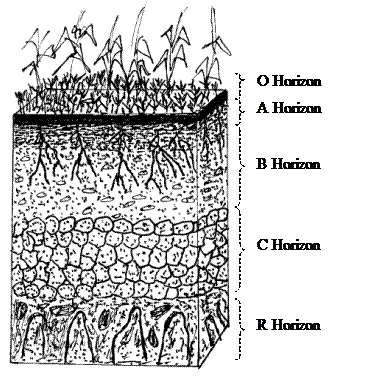
Fig 1: Soil Profile
CONCEPT OF SOIL QUALITY
The soil quality concept evolved throughout the 1990s in response to increased global emphasis on sustainable land use and with a holistic focus emphasizing the sustainable soil management. Soil quality is a concept being developed to characterize the usefulness and health of the soils and depends entirely on the components like soil minerals, organic matter, air, water, and living organisms. This property can change due to human impact on the soil. As soil plays a dominant role in maintaining the ecological balance, it is necessary to maintain the soil quality including soil fertility, potential productivity, resource sustainability and environmental quality (Moen, 1988; Sheppard et al., 1994). In an agricultural context, soil quality is managed, to maximize production without adverse environmental impact, while in a natural ecosystem, soil quality is observed, as a baseline value or set of value against which further change in the system may be compared (Sumner, 1999).
Land use changes modify carbon and litter quality of a location and hence the soil quality (Ojima, 1994). Land cover changes associated with different land use are important agents of environmental change and degradation (Wick et al., 2000).Land cover changes are happening very rapidly in recent times due to over-exploitation and lack of integrated approaches in planning. This is coupled with growing demands of increasing human population. During the past several centuries humans have modified the environmental systems a lot. Most of the world’s forests have been extensively modified by human use of land (Houghton, 1994).This global change in land use has influenced adversely human well being as well as the climate.
The main reason for the change in land use of Western Ghats, the focal area of this study, is the clearing of forest for agricultural purposes (Bhat and Gadgil, 1987), overgrazing, forest fire and land encroachment, etc. Farming in Western Ghats started about three millennia ago but the pre-agricultural phase of vegetation manipulation to promote favored food plants by hunter gatherers could be older. Western Ghats came under first human influences during the Paleolithic or Old Stone Age, over 12,000 years before present (Chandran, 1997). Mesolithic or Middle Stone Age (12,000-5,000 years before present) witnessed the transition of hunter-gatherers into food growers. From this period onwards human beings have an impact on the natural ecosystems. Expansion of traditional agriculture like shifting cultivation in the pre-colonial period and the spread of particularly rubber, coffee and forest tree plantations since the British period beginning two centuries ago, would have wiped out large pockets of primary forests (Chandran, 1997). This has affected the natural property of the soils as well. Many agricultural management practices have detrimental effects on the quantity and quality of soil physico-chemical properties (Caravaca et al., 2002). In addition to reversible land use changes, the soil quality is impaired due to environmental pollution leading to desertification (Sumner, 1999).
In this context, a study has been undertaken to map the soil quality across various land uses in Uttara Kannada district of Central Western Ghats.
The study is undertaken to evolve good soil management practices to suit the central Western Ghats, which form part of a global biodiversity hot spot.
Islam and Weil (2000) studied the changes in biological, chemical and physical properties of soils in response to land use changes and explored relationships among changes in soil properties. Bulk Density varied among cultivated (1.38g/cm3) and natural forest (1.22g/cm3). There was an impact on Water Holding Capacity (WHC) which decreased from 27.9 in natural forest to 22.9 in adjacent cultivated land. Also the cultivated soils were considerably lower in silt and slightly lower in clay than the adjacent soils under natural forest, most likely due to preferential removal of silt by accelerated water erosion during the monsoon month. Compared to natural forest, aggregate stability was lower in cultivated soils, but higher in reforested soils.
Adejuwon and Ekanade (1988) assessed the changes that occur in various soil properties following the removal of forest and cultivation of land to food crops, cocoa and kola. Except for soil texture, all other properties were affected severely. Water holding capacity decreased from forest (60.3%) to cocoa (40.5%). Soil pH differed from 6.8 under forest to slightly acidic under cocoa and kola units. Soil organic matter was 4.7% under forest, 2.6% under fallow, 2.4% under kola and 2.3% under cocoa, while total nitrogen is 0.30% under forest but 0.117% under fallow, and o.15% under both cocoa and kola. Mean values of exchangeable cations viz. Ca, Mg, K and Na are highest under forest and followed by those under fallow. While CEC, is highest under forest i.e. 11.6me/100g while the respective means under fallow, cocoa and kola are 6.4, 5.1 and 4.9me/100g. The study strengthens the point that soil degeneration occurs following the replacement of forest with either tree or arable crops.
Inappropriate land use is one of the main reasons for soil erosion and nutrient loss in the hilly loess area. Bojie Fu et al (2000) studies the effects on soil nutrients like total nitrogen, total phosphorus, available nitrogen, available phosphorus, organic matter and moisture in the land use types in the Yangjuangou catchment of Shaanxi province in China. The study revealed that the forest soil has the most abundant nutrients, with grassland as the second highest and slope farmland the least. In the area which was transformed from forest to slope farmland, total N declined by 64%, available N by 67%, available P by 69% and organic matter by 72% to less than the average values for slope farmland. The 0 – 70 cm soil under forest had the least mean water content (14%), under grassland it was 17% and under slope farmland, 18.5%. Because of the sparse vegetation cover and surface ploughing, the moisture content of slope farmland increased with depth more steeply than under forest or grassland. Except for total and available P, the soil under forest had the highest mean concentration of soil nutrients.
Murty et al (2002) reviewed 20 works to assess changes in soil C upon conversion of forests to agricultural land. The study revealed that bulk density of agricultural soils was 13% more compared to that of natural land use patterns. Soil carbon was the most affected factor since conversion, which reduced 30% from the original land use to cultivation purpose.
When studies estimating carbon and nitrogen were considered, the two decreased 24% and 15% respectively, thus affecting the C: N ratio. It was seen that systems with decreasing N showed increase in C: N ratio, while increasing N showed decrease in C: N ratio. Though there were changes in soil carbon and nitrogen since conversion to pasture from forest, they were not significant.
Land use changes, especially cultivation of deforested land may rapidly diminish soil quality, as ecologically sensitive components of tropical forest ecosystems are not able to buffer the effects of agricultural practices. As a result, severe deterioration in soil quality may lead to permanent degradation of land productivity (Kang and Juo, 1986, Nardi et al, 1996, Islam et al, 1999).
McGrath et al., (2001) reviewed over 100 case studies to understand the effect of land-use change on soil and plant nutrient dynamics in Amazon. Bulk density was significantly higher in pasture than forest due to compaction of soil, which has degraded soil physical properties by decreasing root penetration and consequent water infiltration and gas exchange. This further resulted in the loss of soil organic matter and nutrient runoff. It was found that there is a significant effect on soil pH, bulk density, and exchangeable Ca concentration. Loss of organic carbon (100-300 mg/ha) occur when forests are converted to agricultural lands. Pasture soil have higher total C and N concentration than land use like annual cropping and fallows.
Dinakaran and Krishnayya (2008) studied at Shoolpaneshwar a tropical wildlife sanctuary in Gujarat the influence of different vegetal covers, changes in land use pattern and heterogeneity of physical fractions of the soil organic carbon (SOC) pool on soil carbon. This wildlife sanctuary has some anthropogenic activities, like agriculture by tribal. Soil samples up to 15 cm were collected and analysed for the total carbon. In six sites like Teak plantation, bamboo, mixed vegetation, herbaceous vegetation, paddy field-1 (10 years of cultivation) and paddy field-2 (outside the sanctuary with 50 years of cultivation). The % organic carbon of teak, bamboo, mixed vegetation, herbaceous vegetation, agricultural land-1 and agricultural land-2 are 8.9%, 9.2%, 11%, 2.7%, 3.9% and 2.8% respectively (0.2 cm depth). The paddy field which is continuously used for agriculture shows the lowest organic carbon% than any other land use. The result was that soil organic carbon was much higher in soils with natural tree cover, also the type of vegetal cover has a significant impact on SOC up to a depth of 1.5 m. Changes in land-use pattern severely reduced carbon sink capacity of soils.
Conversion of tropical forests lands to agriculture affects the soil through its effect on soil organic matter and nutrients as well as on physical properties. Brown and Lugo (1990) collected soil samples from mature and secondary forests and agricultural sites in three subtropical life zones of Puerto rico and US virgin islands to determine the effects of forest conversion to agriculture and succession on soil organic carbon and nitrogen contents. In order to prevent the impact of landscape position, sites were selected which were having uniform characteristics like slope, aspect etc. Carbon contents of the cultivated sites, as compared to forest percentage were lower in the wet (44%), moist (31%) than in the dry (86%), while nitrogen was high regardless of the life zone. Conversion of forest to pasture resulted in less soil C and N loss than conversion to crops. They also estimated the succession rate of soil C and N which was found out to be almost same in all the three life zones i.e. 40-50 years for C and 15-20 years for N.
Powers, (2004) studied changes in soil carbon and nitrogen in different land use in Canton in the northern Atlantic lowlands of Costa Rica. Total fifty sites were sampled, and among them 12 were forest to banana transition, 15 pastures to crop transition and four pastures to Vochysis tree plantation transition. The forest to banana plantation transition had the organic Carbon from 6.03% to 4.06%. The conversion of pasture to crop transition decreased the total organic Carbon from 5.4% to 3.63%.The conversion of pasture to Vochysis tree plantation changed soil organic Carbon from 6.79% to 5.49%.The total nitrogen is also decreased from Forest to banana (0.54% to 0.41%), pasture to crop land (0.55% to 0.33%) and pasture to Vochysis tree plantation (0.59% to 0.50%). However, there is an increase in bulk density from forest to banana (0.61g cm -3 to 0.74 g cm -3), pasture to crop land (0.79 g cm -3 to 0.83 g cm -3) and a slight decrease from pasture to Vochysis tree plantation (0.71 g cm -3 to 0.70 g cm -3). Due to rapid cultivation of land, soil losses the organic carbon, total nitrogen pools were also reduced after the conversion of forests to banana plantation.
Effects of land use changes to soil chemical and biochemical properties in Semiarid Mediterranean environment was investigated by Caravaca et al (2002) in four sandy soils under different agricultural practices and four adjacent soils under grass cover. The total organic matter in cultivated soil is 3.2 g Kg-1 and that of native vegetation is 21.3 g Kg-1 indicating a decline of total organic matter due to the conversion of forests to agriculture. General decline of total organic carbon, extractable humic substances (3.6 g Kg-1 to 1.4 g Kg-1), water-soluble carbohydrates (39µg.g1 to 14µg.g1), DHase, urease, protease, phosphatase and ß-glucosidase is noticed in different agricultural practices with respect to soil under grass cover.
Wick et al (2000) investigated soil quality changes associated with the conversion of a native thorn forest (caatinga) into silvo-pastoral systems (buffel grass) in semiarid North East Brazil. Soil nutrients, organic matter, microbial biomass and soil enzymes under native caatinga, preserved native and one introduced tree specieswere compared. The total carbon in caatinga was decreased (14.1 g Kg-1) while converting to buffel grass pasture (12 gKg1).Total nitrogen has declined from 1.1 g Kg-1 (caatinga) to 0.98 g Kg-1 (buffel grass) .Total phosphorus also declined due to conversion of caatinga (176 mg Kg-1) to silvi-pastoral system (173 mg Kg-1). It also decreases the microbial biomass C and Nitrogen. The complete replacement of natural caatinga withsilvo-pastoral system had affected the chemical and biological properties of the soil.
The effect of land use change on soil carbon in forest, pasture, and sugarcane on each end of a rainfall gradient in Hamakua Coast of Hawaii was investigated by Osher et al (2003) Increase in bulk density was noticed in forest (0.38 mg m -2) to pasture (0.44 mg m -2) and then to sugar cane field (0.44 mg m -2) in the first site. In second site also bulk density increases from forest (0.35 mg m -2) to pasture (0.49 mg m -2) to sugar cane field (0.56 mg m -2). There was a decline in organic matter from forest (31.07 kg m-2) to sugar cane field (25.84 kg m-2). Increase in carbon content of pasture due to increased carbon storage in subsurface horizons. Carbon content of the soil under pasture and sugarcane management significantly differs from that of forest in both high rainfall site and low rainfall site. Pasture management results in a net carbon gain and sugar cane land use resulted in a net carbon loss.
Rhoades et al (2000) investigated the soil carbon difference among forest, agricultural and secondary vegetation in western slops of Ecuadorian Andes in tropical lower montane forests. The loss of forest derived soil carbon and the accumulation of carbon from replicate of different land use like sugarcane plantation, Setaria sphacelata pasture, mixed pasture, shrub fallow, and second and old growth forest was studied using stable carbon isotope technique. The result shows that the native forest have a pH of 5 in the top 100 cm. Bulk density increases from 0.67 Mg/m3 in the upper 15 cm to 0.84 Mg/ m3 in lower 15-30 cm (due to the presence of lower organic carbon in the lower 15-30 cm). Soil carbon averages 6.55% to 2.7% in the 0-15 and 15-30 cm depth respectively. In agricultural sites like sugarcane, mixed species and Setaria sphacelata pasture, bulk density was 37%, 32% and 26% more when compared with old-grown forest. They also noticed that sugarcane soils contain 18 Mg/ha less soil C in the upper 15 cm than the native forests (Due to less leaf litter). Mixed species pasture contains 17% less soil carbon than the native forest. This indicates that conversation from forest to crop or pasture has lead to the loss of soil carbon. They also found that continuous production of crop also leads to the reduction of soil carbon.
The effect of soil texture on below ground carbon and nutrient storage in Tapajos National Forest in Brazil of lowland Amazonian forest ecosystem was studied by Silver et al (2004) using field data and the century ecosystem model. Field data includes the total carbon, total nitrogen, texture, and pH, Ca, Mg and K in different depths (0-400 cm). Total organic carbon is 2.81% in 0-50 cm depth but decreased to 2.26 in 350-400 cm depth (due to the presence of low litter quantity in lower horizon). Total Nitrogen is also declining when they sampled from 0-50 cm (0.15%) to 50-150 cm (0.13%). They found a drastic increase in pH while going from 0-50 cm (pH 3.90) to 350-400 (pH 4.19), due to the decrease in humic acid. Increase in percentage of clay (18 % in 0-50 cm depth and 60 % in 350-400 cm depth) and decrease in percentage of sand (80 % in 0-50 cm depth and 38% in 350–400 cm depth) was noticed. Extractable phosphorus, calcium and magnesium also decline from upper horizon to lower horizon. Sandy soil store more carbon of 113 Mg C ha -1 than clay soil of 101 Mg C ha-1 up to 1 M depth. It was found that soil texture play an important role in below ground carbon storage and nutrient availability.
Zhong et al (2000) performed the organic carbon content and distribution in soils under different land uses in tropical and subtropical China, to investigate the C density, stocks and distribution in soils of this region under different land uses by using soil species data from the Second National Soil Survey and the vegetation map of the People’s Republic of China (1:4 M). It was estimated that there is a total of about 28.7±8.2 % organic carbon stored in the upper 1 m of soils of the entire region. Changes in carbon content with depth were observed in the various patterns of land uses. The soils of meadow, herbaceous swamp, and coniferous and broad-leaf forest soils have highest carbon density, while paddy, bush and coppice forest soils showed a low carbon density.
Around the world the regions of immense biodiversity are also the regions which harbour dense human populations. Hence it poses a threat to the natural resources and biodiversity of the region. Due to increasing socio-economic, political and cultural factors, vast tracts of forest lands are brought under cultivation. The soil carbon pool composing of soil organic and soil inorganic carbon is not only critical for soil to perform its productivity and environmental functions, but also plays an important role in global carbon cycle. The sequestration of atmospheric C in soil and biomass not only reduces greenhouse effect but also helps maintain and restore the capacity of soil tom perform its production and environmental functions on sustainable basis (Sahrawat 2003)
The top one metre of the world’s soils contains approximately 1500 Gt C (Johnson & Henderson 1995; Bruce et al. 1999) and even small relative fluxes on a global scale. There is a considerable concern that land use change, in particular, may be leading to a depletion of soil carbon and consequent increases in atmospheric CO2 (IPCC 1997; Bruce et al. 1999, Houghton 1999).
Conversion of tropical forest lands to agriculture affects the soil through its effects on soil organic matter and nutrients as well as on physical properties. Organic matter is an important component of soils because of its influence on cation exchange capacity, soil structure, soil-water balance and a source of plant nutrients (Lal and Kang, 1982; Sanchez, 1976). Losses of organic matter may lead to soil degradation and low crop yields. Understanding the dynamics of soil organic matter and nutrients in tropical forest soils undergoing change and factors influencing these dynamics is important for addressing such issues as the fragility of tropical soils, their sustainability for production and global biogeochemical cycles.
Available studies on tropical soils suggest a rapid decline in organic matter and nitrogen due to continuous cultivation (Allen, 1985; Aweto, 1981; Ayanaba et al., 1976; Brams, 1971; Nye and Greenland, 1964). A recent report (Baker 2007) mentions that less carbon would be taken by Southern oceans than the tropical areas, as previously thought. This re-emphasizes the importance of terrestrial ecosystem in global carbon cycle.
While losses of soil carbon under regular cultivation thus appear to be well established, trends following conversion from forest to pasture have been studied less thoroughly. Lugo and Brown (1993), studied the soil carbon in cultivated and pasture lands, and found that soil carbon is lost under cultivation, but no consistent change in grazed, but uncultivated pastures.
Dr. T.V. Ramachandra
Centre for Sustainable Technologies,
Centre for infrastructure, Sustainable Transportation and Urban Planning (CiSTUP),
Energy & Wetlands Research Group, Centre for Ecological Sciences, Indian Institute of Science, Bangalore – 560 012, INDIA.
E-mail : cestvr@ces.iisc.ernet.in
Tel: 91-080-22933099/23600985,
Fax: 91-080-23601428/23600085
Web: http://ces.iisc.ernet.in/energy
Subash Chandran M.D Energy & Wetlands Research Group, Centre for Ecological Sciences, Indian Institute of Science, Bangalore – 560 012, INDIA.
E-mail:
subhashc@iisc.ac.in
Joshi N.V.Energy & Wetlands Research Group, Centre for Ecological Sciences, Indian Institute of Science, Bangalore – 560 012, INDIA.
E-mail:
nvjoshi@iisc.ac.in
Dhaval Joshi
Energy & Wetlands Research Group, Centre for Ecological Sciences, Indian Institute of Science, Bangalore – 560 012, INDIA.
Maneesh Kumar
Energy & Wetlands Research Group, Centre for Ecological Sciences, Indian Institute of Science, Bangalore – 560 012, INDIA.
Citation: Ramachandra T.V, Subash Chandran M.D, Joshi N.V, Dhaval Joshi, Maneesh Kumar, 2012. Soil Quality across Diverse Landscapes in Central Western Ghats, India , Sahyadri Conservation Series 16, ENVIS Technical Report 42, CES, Indian Institute of Science, Bangalore 560012