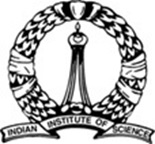 |
Saccharification of macroalgal polysaccharides through prioritized cellulase producing bacteria |
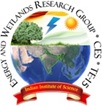 |
Energy and Wetlands Research Group, Centre for Ecological Sciences [CES], Indian Institute of Science, Bangalore – 560012, India.
Web URL: http://ces.iisc.ernet.in/energy; http://ces.iisc.ernet.in/foss
*Corresponding author: Ramachandra T.V Deepthi Hebbale emram.ces@courses.iisc.ac.in, deepthih@iisc.ac.in
|
Introduction
Biofuels produced through contemporary biological processes, rather than geolog- ical processes based fossil fuels (diesel, etc.) are carbon neutral and sustainable, while mitigating greenhouse gas (GHG) footprint in an era of burgeoning oil de- mand and rapidly dwindling stock of fossil fuels (Ramachandra et al., 2009, 2015; Ramachandra and Shwetmala, 2012; Wang et al., 2017). Three main biofuel var-iants are ethanol, biodiesel, and bio-jet fuel. Biofuels are classified into first, sec-ond and third generation based on the feedstock’s carbon source. First generation feedstock was based on the starch based food sources such as wheat, corn, barley etc. The second generation feedstock included biomass rich in lignin and cellulose such as rice straw, wheat straw, sugarcane bagasse etc. Feedstock of the first and second generation received wider resistance due to the conflict of food or land for fuel (Ramachandra et al., 2009). In this context, third generation feedstock involving algae, composed of mostly cellulose and other polysaccharides like starch, laminarin, floridean starch etc. gained significance as feedstock for sustain-able production of biofuel with the reduced greenhouse gas (GHG) footprint. The major constituent of the macroalgal biomass is cellulose, which is a complex chain involving b-1, 4-glycosidic linkages (Kumar and Sahoo, 2012) Conversion of cel- lulose into simple glucose molecule is the major challenge in the ethanol produc- tion (Kumar and Sahoo, 2012; Hebbale et al., 2017; Swain et al., 2017). Algal biomass pretreatment for ethanol production involves reduction in size, loosening of complex structures (e.g. lignin or cellulose fiber). Processes such as steam treat-ment at 200 C, ammonia fibre explosion (AFEX) at moderate temperatures of 90e100 C at high pressure (17e20 bar) and cooking with warm dilute acid have been tried for the release of sugars from the biomass. However, dilute acid pretreatment results in inhibitors such as 5-hydroxymethul furfurals and levulinic acid from sugar degradation, whereas in AFEX little degradation of sugars occurs forming low concentrations of inhibitors (Laureano-Perez et al., 2005; Teymouri et al., 2005; Henning et al., 2012). These inhibitors inhibit subsequent fermenta- tion process. Hence, enzymes are being opted to treat the solid portion (cellulose) of the treated biomass. Enzyme treatment is advantageous due to the lower inhib- itor production (Goldemberg et al., 2007). Algal cell wall is composed of cellulose Ia (triclinic crystalline form) unlike the cellulose Ib (monoclinic crystalline form) in plant cell wall. Cellulose Ia consists of weaker hydrogen bonds resulting from spatial arrangement of individual cellulose chains, resulting in easy access to en- docellulases enzymes during enzyme hydrolysis (Daroch et al., 2013). Conversion of cellulose to simple sugars (glucose) obtained through enzymes is considered as environmentally friendly pretreatment process for biomass conversion. However this research is at nascent stage towards isolating efficient enzyme systems(Swain et al., 2017), and the major challenge is enzyme vary with the feedstock types. Table 1 lists the common enzymes being used in the ethanol production from biomass.
Biotic components of an ecosystem such as animals, plants, bacteria, fungi, aer- obes, anaerobes, mesophiles and thermophiles produce cellulolytic enzymes (Bhat and Bhat, 1997; Niehaus et al., 1999; Zhang and Kim, 2010) in their meta- bolic processes. Bacteria and fungi produce relatively higher extracellular cellu- lase, solubilizing crystalline cellulose. Enzymes secreted by these microorganisms are feasible for large scale production due to their broad biochem- ical diversity, with the possibility of generating mass cultures and also ease of ge-netic manipulation (Zhang and Kim, 2010). Annexure I lists the different sources used in the earlier studies (Mutalik et al., 2012; Erasmus et al., 1997; Hakamada et al., 1997; Li et al., 2003; Verma et al., 2012; Lo et al., 2009; Hussain et al., 2017; Liang et al., 2009; Gupta et al., 2012; Salah et al., 2007; Gao et al., 2008; Ojumu et al., 2003; Bajaj et al., 2003; Liang et al., 2014; Mata et al., 2010; Ventura and Casta~non, 1998; Antonio et al., 2010; Gautam et al., 2011; Bairagi et al., 2002; Dar et al., 2015; Philip et al., 2016; Kamara et al., 2008; Reddy et al., 2003; Krishna, 1999; Dabhi et al., 2014; Maki et al., 2011) about the successful isolation of cellulose degrading bacteria.
Cellulase enzymes are induced by synthetic compounds of disaccharides and thio- cellulose such as palmitate, acetate esters, etc. Conventionally, cellulase is inducedby cellulose itself, where low levels of cellulase from the microbes are produced for hydrolyzing the cellulose to soluble sugars, which are transformed into inducers in the cell and influence DNA binding protein for cellulase gene expression. Bacteria produce mostly endoglucanases that cleave cellulose glyosidic chain at random sites. Synthetic cellulose such as carboxymethyl cellulose (CMC) have been utilized for screening cellulose degrading bacteria. Endoglucanase activity (CMCase) are deter- mined by using soluble substrate like CMC (Percival Zhang et al., 2006), endoglu- canase enzyme cleaves the intermolecular b-1-4-glycosidic bonds present in cellulose. Cellulase enzyme have wide range of potential applications in food, ani- mal feed, textile, fuel, chemical industries, paper industries, waste management (Bhat and Bhat, 1997), etc.
Generation |
Feedstock |
Enzymes |
First generation |
Wheat, Corn, Barley, Rye, Sorghum, Potatoes, Cassava, Sweet potatoes |
a- amylase, Pullulanases Glucoamylases, Amyloglucosidase |
Second generation |
Rice straw, Wheat straw, Sugarcane bagasse Corn stalk, Saw dust, Cotton stalk, Elephant grass, Napier grass, Switch grass |
Endo-b-1,4-glucanase, Cellobiohydrolases, Xylanases b-glucosidases, b-Xylosidases |
Third generation |
Macroalgae |
Cellulase, Carragenase, Agarase |
Table 1. Common enzymes used for biomass conversion for production of bioethanol.
Several diverse bacteria and fungi have been isolated for cellulase enzymes. How- ever, the studies related to isolation of marine organism for the production of cellu- lase enzymes is still in the nascent stages. Marine environment is a large reservoir of microbes with well-developed cellular machinery thriving in extreme conditions like salt concentration of 1.7M, temperature stability of 80e108 C and high pressure of 60MPa (Trivedi et al., 2016). Marine organisms thriving in extreme conditions and tolerance to salts, alkali and other reagents are of particular interest due to the pres- ence of stable enzymes in those microorganisms (Gao et al., 2008). Marine microbial enzymes has potential to offer novel biocatalyst with extraordinary properties (Zhang and Kim, 2010). In this context, the current study focuses on the isolation, identification and characterization of bacteria from different sources for production of cellulase enzyme.
|
Citation : Deepthi Hebbale,R. Bhargavi, T. V.Ramachandra.Saccharification of macroalgalpolysaccharides throughprioritized cellulase producingbacteria.Heliyon 5 (2019) e01372.doi: 10.1016/j.heliyon.2019.e01372
|