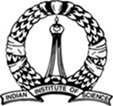 |
Urbanization in India: Patterns,Visualization of Cities, and Greenhouse Gas Inventory for Developing Urban Observatory
|
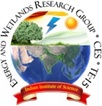 |
aRCGSIDM, Indian Institute of Technology Kharagpur, West Bengal, India bEnergy and Wetland Research Group, Centre for Ecological Science, Indian Institute of Science, Karnataka, India
*Corresponding author: bhaithal@iitkgp.ac.in
Introduction
Urbanization is highly dynamic and now ubiquitous, with almost half the population of the world living in cities. This proportion is expected to reach approximately 72% by 2050 (United Nations 2012). Mega cities now represent the most powerful economic poles of growth and the hinterlands would represent a region with loss of biodiversity and degradation of environment (Czamanski et al. 2008) with increasing human environment and human bioproblems that may range from a micro-region to a local region and can affect global issues. This may include increased air and water pollution (Liu and Diamond 2005), local climate alteration, and increased energy demands(Gonzalez et al. 2005; Ramachandra et al. 2012a). It hasbeen argued that the next phase of urban growth will be concentrated in the developing countries, which includes one of the fastest-growing economies—India (Ramachandra et al. 2012b;Taubenböck et al. 2009, 2012; Van Ginkel 2008).
India has been witnessing rapid urban growth, initially leading to concentrated growth with a steep increase in land prices and later to a fragmented outgrowth from the city boundaries toward the urban–rural side. This fragmented outgrowth often results in the phenomenon called urban sprawl (Bharath et al. 2012; Ramachandra et al. 2015a). Sprawl may be defined as regions and settlements that are located in the vicinity of a city and/or outskirts of the growing urban area with very low to low-density development that would evolve into high-density development in a few years depending on the economy and other major factors. These are connected to the urban core without proper recognition from the city development authorities or planners. These areas are mostly devoid of any basic amenities suas water, transport connectivity, sanitation, and electricity (Ramachandra et al.2014d). Such urban expansions are normally and extensively associated with growth, which is uncoordinated and could result in a serious crisisin terms of sustainable city development and future planning of city growth (Bharath et al. 2013; Ramachandra et al. 2014a,b; Taubenböck et al. 2009).
There is a growing need for landscape monitoring since city managers of mega cities in India face unprecedented challenges with regard to urban planning and land use management owing to the high dynamic growth and change in spatial patterns over time and failures in identifying driving forces and pockets for landscape changes. This necessitates understanding the temporal changes in land use and growth rate, which would help provide vital insights into the decision-making process through understanding the impact of landscape changes, biodiversity, complexity, and fragmentation of the landscape (Bharath et al. 2014; Patino & Duque 2013; Ridd 1995; Zeng & Wu 2005). Therefore, the quantification of landscape changes must consider both modifications in spatial arrangements and their consequences. Simulation-based modeling an provide basic and valuable site-based insights into possible future developments; this includes understanding the paths or pockets of current growth, understanding development corridors attributed to various improving infrastructural facilities, and developmental activities as a result of policy decisions.
Modeling temporal land use dynamics would help in visualizing development scenarios with inputs to sustainable city development aimed at optimizing available resources and decision making (Burgess & Jenks 2002). Urban models based on the principles of cellular automata (CA) are developing rapidly. CA-based urban models usually pay more attention to simulating the dynamic process of urban development and defining the factors or rules driving the development (Batty 2005). Different CA models have been developed to simulate urban growth and urban land use/cover change over time. The differences among various models exist in modifying the five basic elements of CA, that is, the spatial tessellation of cells, states of cells, neighborhood, transition rules, and time (Liu 2009). CA models have been shown to be effective platforms for simulating dynamic spatial interactions among biophysical and socioeconomic factors associated with land use and land cover change (Ramachandra et al. 2012a). Various other approaches, such as regression modeling, neural networks, artificial intelligence, and so on, have been used effectively (Arsanjani et al. 2013; Mozumder & Tripathi 2014; Puertas et al. 2014).
While new urban models have provided insights into urban dynamics, a deeper understanding of the physical and socioeconomic patterns and processes associated with urbanization is still limited in India. Although emerging geospatial techniques have bridged the spatial data gap recently, there remain very few empirical case studies (Thapa & Murayama 2009). The urban population in India is growing at approximately 2.3% per annum, with the global urban population increasing from 13% (220 million in 1900) to 49% (3.2 billion in 2005) and is projected to escalate to 60% (4.9 billion) by 2030 (Ramachandra & Kumar 2008). The increase in urban population in response to the growth in urban areas is mainly attributed to migration. There are 48 urban agglomerations/cities having a population of more than 1 million in India (in 2011). However, unplanned urbanization coupled with the lack of holistic approaches leads to the lack of infrastructure and basic amenities. Hence, proper urban planning with operational, developmental, and restorative strategies is required to ensure the sustainable management of natural resources. Unplanned growth would involve radical land use conversion of forests, surface water bodies, and so on with the irretrievable loss of ground prospects (Basawaraja et al. 2011). The process of urbanization could be either in the form of townships or unplanned or organic. Many organic towns in India are now influencing large-scale infrastructure development and so on as a result of the impetus from the national government through development schemes such as JNNURM (Jawaharlal Nehru National Urban Renewal Mission). The focus is on the fast-track development through efficient infrastructure and delivery mechanisms, community participation, and so on. Large-scale land use and land cover changes, such as the loss of forests to meet the urban demands of fuel and land (Ramachandra & Kumar 2009), have led to changes in ecosystem structure, affecting its functioning and thereby threatening sustainable development. Cities are often attributed as the engines of economic growth. This compels cities to become smarter in handling large-scale urbanization and in finding new ways to manage complexity, increase efficiency, and improve quality of life. The design of smart cities requires an understanding of spatial patterns of urbanization to implement appropriate mitigation measures. This necessitates spatial information for the city administrators to visualize the patterns of urbanization and also predict the likely changes with the implementation of decisions (such as “what if” scenarios).Smart cities would be self-reliant and self-sufficient systems, providing basic necessities (such as water, sanitation, reliable utility services, and health care), transparent government transactions, and various citizen-centric services. This leads to the first objective of this chapter, that is, to understand the land use changes in five major cities in India.
1.1 Carbon Footprint Analysis
India has been transitioning from a dependent economy to a more self-reliant manufacturing economy.This essentially involves the manufacturing process, which would entail more greenhouse gas (GHG)emissions, and a large workforce, which would increase the amount of carbon in the atmosphere. Such a transition to suit a very low carbon economy entails the adoption of modern technologies and adaptation strategies that involve improved regulatory mechanisms, better infrastructures, best business practices, low consumption rates, and improved green lifestyles. It should be noted that in the past two decades, an increased concern about global warming has been raised because of the increased emission of GHGs that form a blanket in the atmosphere, with all countries focusing on efforts to minimize these emissions. There has been a large-scale change in industrial output through the transformation of heavy industries in developed countries to a more knowledge-based and service-based economy that is comparatively cleaner (Shafik and Bandyopadhyay 1992). It must also be noted that there have been active citizen participation and awareness campaigns worldwide that have led to improved environmental regulations, which, in turn, decreased the rate of environmental degradation. Given these, international governing bodies should set targets to accomplish tasks and solve global environmental problems.
Also, organizations that govern environmental regulations are trying to establish strategies that would help mitigate GHGs of anthropogenic origin, thus reducing the threat of global warming (Kennedy et al. 2010; Wiedmann & Minx 2008). This resulted in many metropolitan cities across the globe showing interest in estimating GHG emissions and developing strategies to reduce emissions. Thus, carbon footprint is a measure of the impact of human activities on the environment in terms of the amount of GHGs produced (Finkbeiner 2009; Ramachandra et al. 2017). Carbon footprint, also called GHG footprint through estimation of GHG emission, is expressed in terms of carbon dioxide equivalent, indicating the amount of carbon in the atmosphere of a particular region. Carbon dioxide equivalent(CO2 e) is a unit for comparing the radiative forcing of a GHG (measure of influence of a climatic factor in changing the balance of energy radiation in the atmosphere) to that of carbon dioxide (ISO 2006a,b, Ramachandra et al. 2017). It is the amount of carbon dioxide by weight that is emitted into the atmosphere that would produce the same estimated radiative forcing as a given weight of another radiatively active gas (Wiedmann and Minx 2008). 2
1.2 Necessity of Understanding Carbon Footprint of a Region through Quantification
Climate change is now one of most concerning areas for human existence in the next century. Decreasing our carbon footprint has the potential to reduce its impact on climate change by increasing human comfort. It would also add to the valuable information required as a component for sustainable urban planning for planners, policy makers, and local municipalities (Courchene and Allan 2008).
It has been widely reported that the last four decades have witnessed an abrupt increase in concentration of GHGs. All human activities such as industry, agriculture, deforestation, waste disposal, and specifically the burning of fossil fuels for various applications are considered the main factors that contribute to the increase in fossil fuel content in the atmosphere. The concentrations of atmospheric CO2 increased from approximately 80 ppm considered by volume (ppmv) in the 1950s to 372 ppmv in 2001 and has been increasing approximately 20 ppmv every year as per the 2007 IPCC report. Similarly, CH4 emissions have increased by 0.02 ppmv. This rapid increase in GHG concentrations in the atmosphere, mainly due to anthropogenic activities of humans, has affected the local and global climate and is said to have farreaching consequences. The most significant contributors to global warming are the six Kyoto gase and, to a lesser extent, the chlorofluorocarbons (highlighted during the Montreal protocol; IPCC 2006, 2007). CO2is the primary GHG (contributing ~60%) followed by methane and nitrous oxide, with the largest GHG-producing sector being the energy supply sector (~26%) (IPCC 1996, 2007).
The effect of these gases is measured in terms of global warming potential (GWP) and depends on radiative forcing and time frame (usually 100 years). The GWP factor for CO2 is 1 (considered as reference), that for CH 4is 21, and that for N2O is 298 (IPCC 2007). Today, it is important to understand and quantify the GHG emissions through a sectorial approach with specific methods. A sectorial approach would help in planning sectorial interventions in reducing carbon footprint in the atmosphere as well as necessary technological interventions based on sectorial needs.
Given these requirements, this chapter addresses three basic objectives based on understanding the steps in building and transforming sustainable urban systems. The first objective is to understand temporal land use change in the last two decades in five major cities in India and to model it based on agents of change. The second objective is to quantify GHG emissions. The third objective is to understand policy interventions required to overcome both land use change effects and effects of GHG emissions.
Citation : Bharath Haridas Aithal, Mysore Chandrashekar Chandan, Shivamurthy Vinay, T.V. Ramachandra, 2018, Urbanization in India: Patterns, Visualization of Cities, and Greenhouse Gas Inventory for Developing Urban Observatory. CRC Press Taylor & Francis Group 6000 Broken Sound Parkway NW, Suite 300 Boca Raton, FL 33487-2742 © 2018 by Taylor & Francis Group, LLC CRC Press is an imprint of Taylor & Francis Group, an Informa business.
* Corresponding Author : |
|
Bharath Haridas Aithal
RCGSIDM, Indian Institute of Technology Kharagpur, West Bengal, India
Energy and Wetland Research Group, Centre for Ecological Science, Indian Institute of Science, Karnataka, India
E-mail : bhaithal@iitkgp.ac.in |
|
|
Bharath Haridas Aithal
aRCGSIDM, Indian Institute of Technology Kharagpur, West Bengal, India
bEnergy and Wetland Research Group, Centre for Ecological Science, Indian Institute of Science, Karnataka, India
* Corresponding Author
E-mail : bhaithal@iitkgp.ac.in
Mysore Chandrashekar Chandan aRCGSIDM, Indian Institute of Technology Kharagpur, West Bengal, India
Citation:Bharath Haridas Aithal, Mysore Chandrashekar Chandan, Shivamurthy Vinay, T.V. Ramachandra, 2018, Urbanization in India: Patterns, Visualization of Cities, and Greenhouse Gas Inventory for Developing Urban Observatory. CRC Press Taylor & Francis Group 6000 Broken Sound Parkway NW, Suite 300 Boca Raton, FL 33487-2742 © 2018 by Taylor & Francis Group, LLC CRC Press is an imprint of Taylor & Francis Group, an Informa business.
Shivamurthy Vinay bEnergy and Wetland Research Group, Centre for Ecological Science, Indian Institute of Science, Karnataka, India
|