Introduction
Greenhouse gas (GHG) levels in the atmosphere have increased during the post industrialization era by 25% and estimates reveal that about three-quarters anthropogenic carbon-dioxide are due to burning of fossil fuels. Fast dwindling stocks of fossil fuels and consequences of GHG emissions have necessitated the exploration of cost effective sustainable energy sources [1,2,3]. Estimates reveal that fossil fuels such as oil, coal and gas would deplete in 35, 107 and 37 years respectively [4], highlighting the impending energy crisis and the escalation of fuel prices with the dwindling of natural resources stock. Apart from this, nutrient accumulations in ecosystems due to indiscriminate disposal of liquid wastes have further enhanced GHG levels posing the threat of global warming and consequent changes in the climate.
Energy demand has increased with the spurt in economic activities, bringing along a change in the consumption pattern, which in turn varies with the source and availability of its energy, conversion loss and end use efficiency. The growing demand of burgeoning population coupled with developmental activities based on ad-hoc decisions have led to resource scarcity in many parts of India. In this context studies have shown that algae during their growth process synthesize carbohydrates during photosynthesis and then stock lipids variably. They act as a solar energy driven cell factory [5] converting CO2 to O2 thereby reducing the atmospheric CO2 while trapping nutrients from the environment. Cleaner, sustainable and cost-effective energy alternatives can be accomplished through the tiny oleaginous microorganisms like algae. Microalgae based biofuel are capable of meeting the global demand of fuels [5,6] with higher efficiency to overcome global warming, due to their higher growth rate requiring lesser cultivation area and higher biomass production [7]. Algae have good prospects as biofuel feedstock due to the higher photosynthetic efficiency, continuous year round productions and the ability to thrive in municipal wastewaters, marginal lands, etc. Figure 1 compares the oil yield potential of various food crops with microalgae [1, 8]. It is apparent that algae have the potential to produce up to ten times more oil per hectare land than other traditional biofuel crops like Elaeis oleifera (oil palm), Jatropha curcas (jatropha), (Glycine max) soybean etc. [1, 8, 9].
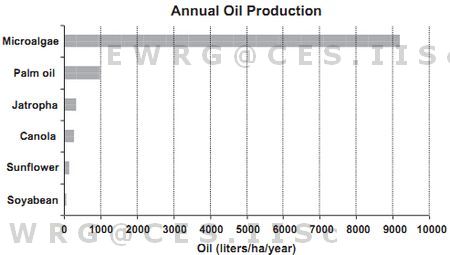
Figure 1: Potential oil yield per hectare per year (adapted from Emily, 2009)
India meets about 75-80% of its total petroleum requirements through imports [10] causing a heavy burden on the foreign exchange. This has necessitated exploration for viable alternatives to diesel, for meeting the demand of transport, industrial and agricultural sectors [11]. The transportation sector in India consumes almost five times more diesel fuel than gasoline compared to other countries [9]. The annual consumption of diesel and gasoline from July 2011 to July 2012 is about 5.735 and 1.321 Million tonnes (Mt) respectively [12]. Diesel consumption has increased by almost two-folds in the last two decades [9]. This highlights the need for cost effective sustainable indigenous alternative to ensure food and fuel security in India. Moreover most of the urban municipalities are unable to handle wastewaters due to rapid urbanisation and lack of appropriate infrastructure. Wastewater generated in most urban areas is either untreated or partially treated and are let into nearby water-bodies. Wastewaters rich in C, N and P enhances the nutrient levels in the receiving water bodies (such as tanks, lakes and rivers) leading to eutrophication [13]. Conventional treatment plants (primary and secondary) are unable to remove nutrients particularly N and P. In this context, lagoons or algal ponds are attractive and viable solution for treating wastewater to the accepted levels [14, 15]. Furthermore the sustained availability of nutrients through wastewaters allows prolific growth of micro algae, which can be harvested for extracting lipids. This would help in cost effective wastewater treatment at local levels, while giving an opportunity for clean energy production.
Many species of algae grow copiously in wastewater utilising abundantly available organic carbon and inorganic nutrients (N and P) and hence play an important remediation role in efficient removal of N and P [16, 17]. The use of algae in wastewater treatment has been in practice since long [18] through the use of conventional oxidation (stabilization) ponds or the suspended algal pond systems (such as high-rate algal ponds HRAP) which have been highly effective [19, 20]. In Algal systems, a significant amount of O2 generation from photosynthetic algae helps in aeration avoiding mechanical aeration of the treatment pond and additional associated operational costs [17]. Oxygenation of ponds through algae also aids in bioremediation of organic and inorganic compounds by heterotrophic aerobic bacteria [21]. Furthermore, algal based remediation is environmentally amenable and sustainable as it does not generate additional pollutants such as sludge by-products and provides an opportunity for efficient recycling of nutrients.
Most of the research on algal wastewater treatment is based on the analysis of laboratory-based small scale and pilot pond-scale cultures and outdoor large open ponds [18, 19]. The studies investigating the growth pattern of algae under a variety of wastewater conditions have focussed on evaluating the potential of algae for removing N and P, and in some instances metals from wastewater [16]. However the information pertaining to the potential of wastewater algae as an alternative energy options is scant. Experimental studies addressing the factors to maximise algal biomass production and harvesting will benefit the evaluation of wastewater-grown algae as a potential biofuel option.
The inadequacy of fossil fuels and increasing oil prices along with the increasing levels of greenhouse gas emissions poses inevitable threats to global environment, and necessitates our dependencies on alternative energy sources as algal fuel [1]. The concept of algal usage as biofuel feedstock, followed by lipid extraction and transesterification have recently been realised as the step towards exploring sustainable options for meeting the energy demand [9]. Steady-state nutrient supply and ways of extracting lipid are the most challenging stages in realising algae based biofuel [22]. The algal biofuel production involves a series of unit processes including species selection, cultivation, biomass harvesting, and lipid extraction. This requires a sound understanding of algal downstream processing with the process optimisation for successful commercial exploitation.
Cell disruption is the most crucial step for recovering intracellular products from algae that enhances the lipid extraction efficiency [23]. The efficiency of the disruption methods depends upon the ways to lyse the targeted cell by effectively breaking the cell wall components. Cell disruption can be done through mechanical, physical, chemical, enzymatic or combined methods. Various cell disruption techniques include bead beating, sonication, microwave, high-pressure homogenisers, autoclaving and addition of hydrochloric acid, sodium hydroxide, or alkaline lysis [24,25]. The selection of solvents for extracting metabolites as fatty acids is essential as it causes alterations to the cell membrane to enhance the movement of globules out of the algal cells [26]. Thus the bio-chemical properties of cell walls play a vital role in the solvent extraction process. Generally a thick cell wall is commonly found in microalgae rendering them suitable to survive in harsh conditions. The characteristics of algae plays an important role for lipid recovery, that would be from cells, which are strong enough to withstand shear stresses at the same time, can lyse easily to obtain a reasonable lipid yield.
Earlier studies indicate that bead beating is the most effective cell disruption method for Botryococcus braunii [27]. Nevertheless bead beating induces direct mechanical damage to the cell as they are subjected to high stress produced by high pressure, abrasion during rapid agitation by glass beads [28]. Bead beating has been suggested for cell disruption with good potential for industrial scale up [23]. However sonication is one of the most widely used laboratory disruption technique, where high acoustic power inputs (sound waves of frequency higher than 15-20 kHz) are used to disrupt the cell wall [28]. Sonication is said to be the most applicable and efficient method of lipid extraction from microalgae [25].
The objective of the present work is to explore the lipid prospects from microalgae of urban wastewater ponds, which involves:
- The analysis of conditions required for higher algal growth in municipal wastewater;
- determination of algal lipid potential in urban wastewaters;
- comparative analysis of different cell disruption methods to find the best method for lipid extraction for improved yield of essential fatty acids present in three different wastewater grown algal species; and
- analysis of composition of fatty acids in selected algal species and their suitability as biofuel.
|