MECHANISMS
Biosorption Mechanisms: The complex structure of microorganisms implies that there are many ways for the metal to be taken up by the microbial cell. The biosorption mechanisms are various and are not fully understood. They may be classified according to various criteria.
According to the dependence on the cell's metabolism, biosorption mechanisms can be divided into:
- Metabolism dependent and
- Non -metabolism dependent.
According to the location where the metal removed from solution is found, biosorption can be classified as
- Extra cellular accumulation/ precipitation
- Cell surface sorption/ precipitation and
- Intracellular accumulation.
Transport of the metal across the cell membrane yields intracellular accumulation, which is dependent on the cell's metabolism. This means that this kind of biosorption may take place only with viable cells. It is often associated with an active defense system of the microorganism, which reacts in the presence of toxic metal.
During non-metabolism dependent biosorption, metal uptake is by physico-chemical interaction between the metal and the functional groups present on the microbial cell surface. This is based on physical adsorption, ion exchange and chemical sorption, which is not dependent on the cells' metabolism. Cell walls of microbial biomass, mainly composed of polysaccharides, proteins and lipids have abundant metal binding groups such as carboxyl, sulphate, phosphate and amino groups. This type of biosorption, i.e., non-metabolism dependent is relatively rapid and can be reversible (Kuyucak and Volesky, 1988).
In the case of precipitation, the metal uptake may take place both in the solution and on the cell surface (Ercole, et al. 1994). Further, it may be dependent on the cell's' metabolism if, in the presence of toxic metals, the microorganism produces compounds that favour the precipitation process. Precipitation may not be dependent on the cells' metabolism, if it occurs after a chemical interaction between the metal and cell surface.
Transport across cell membrane: Heavy metal transport across microbial cell membranes may be mediated by the same mechanism used to convey metabolically important ions such as potassium, magnesium and sodium. The metal transport systems may become confused by the presence of heavy metal ions of the same charge and ionic radius associated with essential ions. This kind of mechanism is not associated with metabolic activity. Basically biosorption by living organisms comprises of two steps. First, a metabolism independent binding where the metals are bound to the cell walls and second, metabolism dependent intracellular uptake, whereby metal ions are transported across the cell membrane. ( Costa, et.al., 1990, Gadd et.al., 1988, Ghourdon et.al., 1990, Huang et.al., 1990., Nourbaksh et.al., 1994)
Physical adsorption: In this category, physical adsorption takes place with the help of van der Waals' forces. Kuyucak and Volesky 1988, hypothesized that uranium, cadmium, zinc, copper and cobalt biosorption by dead biomasses of algae, fungi and yeasts takes place through electrostatic interactions between the metal ions in solutions and cell walls of microbial cells. Electrostatic interactions have been demonstrated to be responsible for copper biosorption by bacterium Zoogloea ramigera and alga Chiarella vulgaris (Aksu et al. 1992), for chromium biosorption by fungi Ganoderma lucidum and Aspergillus niger .
Ion Exchange: Cell walls of microorganisms contain polysaccharides and bivalent metal ions exchange with the counter ions of the polysaccharides. For example, the alginates of marine algae occur as salts of K+, Na+, Ca2+, and Mg2+. These ions can exchange with counter ions such as CO2+, Cu2+, Cd2+ and Zn2+ resulting in the biosorptive uptake of heavy metals (Kuyucak and Volesky 1988). The biosorption of copper by fungi Ganoderma lucidium (Muraleedharan and Venkobachr, 1990) and Aspergillus niger was also up taken by ion exchange mechanism.
Complexation: The metal removal from solution may also take place by complex formation on the cell surface after the interaction between the metal and the active groups. Aksu et al. 1992 hypothesized that biosorption of copper by C. vulgaris and Z. ramigera takes place through both adsorption and formation of coordination bonds between metals and amino and carboxyl groups of cell wall polysaccharides. Complexation was found to be the only mechanism responsible for calcium, magnesium, cadmium, zinc, copper and mercury accumulation by Pseudomonas syringae. Microorganisms may also produce organic acids (e.g., citric, oxalic, gluonic, fumaric, lactic and malic acids), which may chelate toxic metals resulting in the formation of metallo-organic molecules. These organic acids help in the solubilisation of metal compounds and their leaching from their surfaces. Metals may be biosorbed or complexed by carboxyl groups found in microbial polysaccharides and other polymers.
Precipitation: Precipitation may be either dependent on the cellular metabolism or independent of it. In the former case, the metal removal from solution is often associated with active defense system of the microorganisms. They react in the presence of a toxic metal producing compounds, which favour the precipitation process. In the case of precipitation not dependent on the cellular metabolism, it may be a consequence of the chemical interaction between the metal and the cell surface. The various biosorption mechanisms mentioned above can take place simultaneously.
Use of Recombinant bacteria for metal removal: Metal removal by adsorbents from water and wastewater is strongly influenced by physico-chemical parameters such as ionic strength, pH and the concentration of competing organic and inorganic compounds. Recombinant bacteria are being investigated for removing specific metals from contaminated water. For example a genetically engineered E.coli, which expresses Hg2+ transport system and metallothionin (a metal binding protein) was able to selectively accumulate 8 mmole Hg2+/g cell dry weight. The presence of chelating agents Na+, Mg2+ and Ca2+ did not affect bioaccumulation.
Factors affecting Biosorption : The investigation of the efficacy of the metal uptake by the microbial biomass is essential for the industrial application of biosorption, as it gives information about the equilibrium of the process which is necessary for the design of the equipment.
The metal uptake is usually measured by the parameter 'q' which indicates the milligrams of metal accumulated per gram of biosorbent material and 'qH' is reported as a function of metal accumulated, sorbent material used and operating conditions.
The following factors affect the biosorption process:
1. Temperature seems not to influence the biosorption performances in the range of 20-35 0C (Aksu et al. 1992)
2. pH seems to be the most important parameter in the biosorptive process: it affects the solution chemistry of the metals, the activity of the functional groups in the biomass and the competition of metallic ions (Friis and Myers-Keith, 1986, Galun et al. 1987)
3. Biomass concentration in solution seems to influence the specific uptake: for lower values of biomass concentrations there is an increase in the specific uptake (Fourest and Roux, 1992; Gadd et al. 1988). Gadd et al. 1988 suggested that an increase in biomass concentration leads to interference between the binding sites. Fourest and Roux, 1992 invalidated this hypothesis attributing the responsibility of the specific uptake decrease to metal concentration shortage in solution. Hence this factor needs to be taken into consideration in any application of microbial biomass as biosorbent.
4. Biosorption is mainly used to treat wastewater where more than one type of metal ions would be present; the removal of one metal ion may be influenced by the presence of other metal ions. For example: Uranium uptake by biomass of bacteria, fungi and yeasts was not affected by the presence of manganese, cobalt, copper, cadmium, mercury and lead in solution (Sakaguchi and Nakajima, 1991). In contrast, the presence of Fe2+ and Zn2+ was found to influence uranium uptake by Rhizopus arrhizus (Tsezos and Volesky, 1982) and cobalt uptake by different microorganisms seemed to be completely inhibited by the presence of uranium, lead, mercury and copper (Sakaguchi and Nakajima, 1991).
Biosorption equilibrium models - Assessment of sorption performance: Examination and preliminary testing of solidliquid sorption system are based on two types of investigations: (a) equilibrium batch sorption tests and (b) dynamic continuous flow sorption studies.
The equilibrium of the biosorption process is often described by fitting the experimental points with models (Gadd, et al. 1988) usually used for the representation of isotherm adsorption equilibrium. The two widely accepted and linearised equilibrium adsorption isotherm models for single solute system are given by the following:
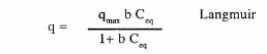
where q is milligrams of metal accumulated per gram of the biosorbent material; Ceq is the metal residual concentration in solution; qmax is the maximum specific uptake corresponding to the site saturation and b is the ratio of adsorption and desorption rates. This is a theoretical model for monolayer adsorption.
Another empirical model for monolayer adsorption is
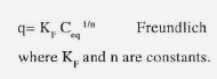
These models can be applied at a constant pH. These models are used in literature for modeling of biosorption equilibrium in the presence of one metal. These values are plotted in a 2D line where the specific uptake q is reported as a function of the metal concentration Ceq
But the above said adsorption isotherms may exhibit an irregular pattern due to the complex nature of both the sorbent material and its varied multiple active sites, as well as the complex solution chemistry of some metallic compounds (Volesky and Holan, 1995). Evaluation of equilibrium sorption performance needs to be supplemented by process-oriented studies of its kinetics and eventually by dynamic continuous flow tests.
Biosorption by immobilized cells: Microbial biomass consists of small particles with low density, poor mechanical strength and little rigidity. The immobilization of the biomass in solid structures Qeates a material with the right size, mechanical strength and rigidity and porosity necessary for metal accumulation. Immobilisation can also yield beads and granules that can be stripped of metals, reactivated and reused in a manner similar to ion exchange resins and activated carbon.
Various applications are available for biomass immobilization. The principal techniques that are available in literature for the application of biosorption are based on adsorption on inert supports, on entrapment in polymeric matrix, on covalent bonds in vector compounds, or on cell cross-linking.
Adsorption on inert supports: Support materials are introduced prior to sterilization and inoculation with starter culture and are left inside the continuous culture for a period oftime, after which a film of microorganisms is apparent on the support surfaces. This technique has been used by Zhou and Kiff, 1991 for the immobilization of Rhizopus arrhizus fungal biomass in reticulated foam biomass support particles; Macaskie et al. 1987, immobilised the bacterium Citrobacter sp. by this technique. Scott and Karanjakar 1992, used activated carbon as a support for Enterobacter aerogens biofilm. Bai and Abraham, 2003 immobilized Rhizopus nigricans on polyurethane foam cubes and coconut fibres.
Entrapment in polymeric matrices: The polymers used are calcium alginate (Babu et al. 1993, Costa and Leite, 1991, Peng and Koon, 1993, Gulay Bayramoglu et al. 2002), polyacrylamide (Macaskie et aI., 1987, Michel et al. 1986, Sakaguchi and Nakajima et al. 1991, Wong and Kwok, 1992), polysulfone (Jeffers et al. 1991, Bai and Abraham, 2003) and polyethylenimine (Brierley and Brierley, 1993). The materials obtained from immobilization in calcium alginate and polyacrylamide are in the form of gel particles. Those obtained from immobilization in polysulfone and polyethyleneimine are the strongest.
Covalent bonds to vector compounds: The most common vector compound (carrier) is silica gel. The material obtained is in the form of gel particles. This technique is mainly used for algal immobilization (Holan et al. 1993, Mah:!mn and Holocombe, 1992).
Cross-linking: The addition of the cross-linker leads to the formation of stable cellular aggregates. This technique was found useful for the immobilization of algae (Holan et al. 1993). The most common cross linkers are: formaldehyde, glutaric dialdehyde, divinylsulfone and formaldehyde - urea mixtures.
Desorption: If the biosorption process were to be used as an alternative to the wastewater treatment scheme, the regeneration of the biosorbent may be crucially important for keeping the process costs down and in opening the possibility of recovering the metals extracted from the liquid phase. For this purpose it is desirable to desorb the sorbed metals and to regenerate the biosorbent material for another cycle of application. The desorption process should:
• yield the metals in a concentrated form;
• restore the biosorbent to close to the original condition for effective reuse with undiminished metal uptake and
• no physical changes or damage to the biosorbent.
While the regeneration of the biosorbent may be accomplished by washing the metal- laden biosorbent with an appropriate solution, the type and strength of this solution would depend on the extent of binding of the deposited metal. Dilute solutions of mineral acids like hydrochloric acid, sulphuric acid, acetic acid and nitric acid can be used for metal desorption from the biomass (de Rome and Gadd, 1987, Zhou and Kiff, 1991, Luef et.al. 1991, Holan et.al. 1993, Pagnanelli etal. 2002, Baiand Abraham, 2003).
Polysulphone immobilized Rhizopus nigricans were subjected to Cr (VI) recovery experiments using 0.01 N solutions of mineral acids, salt solutions, alkalies, deionised distilled water and buffer solutions. The percentage desorption by various eluants is given in Table 2.
A few experiments were conducted to desorb the metal ions from the loaded waste fungal biomass of Aspergillus species (Chandrashekar et al. 1998) as a function of HCl concentration in the case of iron, calcium and nickel. The results revealed that with increase in HCl concentrations, the desorption of the metal ions increased and at 5M HCI, complete removal of calcium and iron would be achieved while about 78% Nickel would be desorbed.
The desorption of the adsorbed Hg (II) from the biosorbent - immobilized and heat inactivated Trametes versicolor and Pleurotus sajur-caju were studied in a batch system (Arica et al. 2003). Hg (II) ions adsorbed onto the biosorbents were eluted with 10 mmol dm-3 HCl and the results showed that more than 97% of the adsorbed Hg (II) ions were desorbed from the biosorbents.
Effect of Pre-treatment on the biosorption of heavy metals: Metal affinity to the biomass can be manipulated by pretreating the biomass with alkalies, acids, detergents and heat, which may increase the amount of the metal sorbed. The bioadsorption capacity of autoclaved Mucor rouxii decreased as compared to the live fungus, attributed to the loss of intracellular uptake (Yan and Viraraghavan, 2000). Whistler and Daniel (1985) reported that the heat treatment could cause a loss of amino-functional groups on the fungal surface through the non-enzymic browning reaction. Aminofunctional groups in the polysaccharides contribute to the binding of heavy metals (Loaec et al., 1997). However, Galun et al., 1987 reported that Pencillium biomass pretreatment at 100°C for 5 minutes increased the biadsorption of lead, cadmium, nickel and zinc and the increase was attributed to the exposure of latent binding sites after pre-treatment.
In the case of alkali pre-treatment, bioadsorption capacity of Mucor rouxii biomass was significantly enhanced in comparison with autoclaving (Yan and Viraraghavan, 2000). In a study by Galun et al. (1987), NaOH treated Pencillium digitatum also showed enhancement of cadmium, nickel and zinc biosorption. Removal of surface impurities, rupture of cell-membrane and exposure of available binding sites for metal bioadsorption after pre-treatment may be the reason for the increase in metal bioadsorption. McGahren et al. (1984), Brierly et.al (1985) and Muraleedharan and Venkobachar (1990) showed that alkali treatment of biomass may destroy autolytic enzymes that cause putrefaction of biomass and remove lipids and proteins that mask reactive sites. The cell wall of Mucor rouxii was ruptured by NaOH treatment. Besides, the pre-treatment could release polymers such as polysaccharides that have a high affinity towards certain metal ions (Mittelman and Geesey, 1985; Loaec et.al. 1997).
Acid pretreatment of Mucor rouxii significantly decreased the bioadsorption of heavy metals (Yan and Viraraghavan, 2000), which is in agreement with the observation of Kapoor and Viraraghvan (1998) in the case of A.niger. This is attributed to the binding ofH+ ions to the biomass after acid treatment may be responsible for the reduction in adsorption of heavy metals. The polymeric structure of biomass surface exhibits a negative charge due to the ionization of organic and inorganic groups (Hughes and Poole, 1989). Bux and Kasan (1994) suggested that the higher the biomass electronegativity, the greater the attraction and adsorption of heavy metal cations. Thus the remaining H+ions on the acidic pretreated M.rouxii biomass may change the biomass electronegativity, resulting in a reduction in bioadsorption capacity.
However, Huang and Huang (1996) reported that acid pretreatment can strongly enhance the adsorption capacity of Aspergillus.oryzae mycelia. In case of A.oryzae, live biomass after acid pre-treatment was directly used in bioadsorption of heavy metals instead of being autoc1aved and dried. The difference in results after a specific pretreatment may be attributed to the different strains of fungi used and whether the biomass was live or stead when it is used in biosorption of metal ions. For example; pre-treatment of A.oryzae by HClO4 increased the bioadsorption of lead, cadmium and nickel, but it was not the case for the species of R.oryzae (Huang and Huang, 1996). When non-viable biomass is used in the removal of heavy metals, alkali pretreatment is an effective method to improve the bioadsorption capacity for metal ions (Yan and Viraraghavan, 2000). Hence, the bioadsorption efficiency of dead biomass may be greater, equivalent to, or less than that of live biomass depending on the pre-treatment method applied. It is necessary to carry out more detailed studies to understand why enhancement or reduction in adsorption capacity occurs under specific pre-treatment conditions.
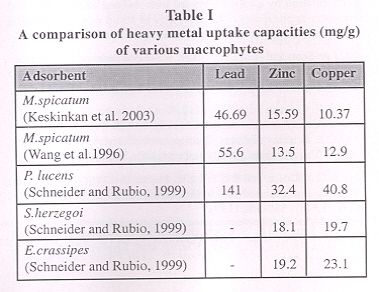
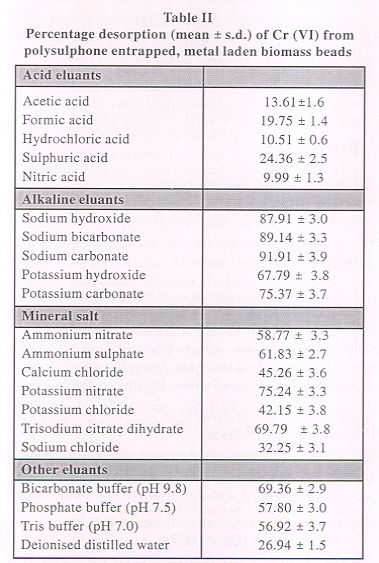
|