Results and Discussion
Variations in Hydrological Parameters
Figure 4.a-d reflects the variability of physical parameters (AT,
WT, pH, and Salinity) at sampling locations. Temperature is a
critical physical factor that controls the rate of
photosynthesis in addition to influencing many other chemical
and biological reactions (Fatema et al., 2014). Temperature
variations are influenced by prevailing precipitation and
humidity levels. In this study, air temperature during
pre-monsoon season was varying across stations with a mean
temperature of (av. 31.36 ± 1.20 oC). During
premonsoon, the estuary was characterized by warm (31.42 ± 0.66
oC) with high saline (30.20 ± 3.45 ppt) water in all
stations (S1 to S6): lower (stations S5, S6), central (stations
S3, S4) and upper reaches (stations S1, S2).
During monsoon season, the estuary had relatively lower
variations of air and surface water temperatures (~ 1 to 3 oC),
with air temperature of (29.06 ± 0.67 oC) and water
temperature (28.11 ± 0.87 oC) respectively. The mean
air and water temperature across the stations during
post-monsoon season was (31.73 ± 0.82 oC) and (29.04
± 0.56 oC). This drop-in water temperature during
post-monsoon season could be attributed to heavy precipitation
that resulted in the inflow of cold freshwater from the Ghats.
Generally, in a tropical estuary, the temperature is inversely
correlated with salinity and water transparency (Fatema et al.,
2014).
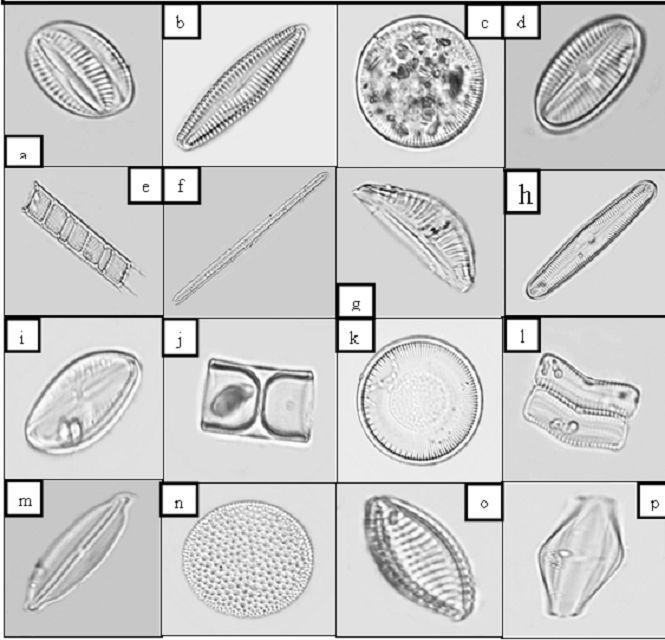
Figure 3 Light Micrographs of Select Diatom
Species a) Navicula forcipata b) Gomphonema
sp. c) Cyclotella operculata d) Diploneis
subovalis e) Aulacoseira granulata f) Bacillaria
paradoxa g) Epithema gibberula h) Pinnularia
sp. i) Navicula integra j) Melosira
jurgensii k) Cyclotella meneghiniana l) Achnanthes
brevipes m) Frustulia crassinervia n) Coscinodiscus
radiatus o) Raphoneis amphiceros p) Amphora
salina
The water quality results depicted the seasonal
variability. Air temperature showed wider fluctuations primarily
due to higher insolation during pre-monsoon seasons followed by
reduced levels due to precipitation. Air and water temperature
variations were comparable to earlier studies in tropical
estuaries of the south-west coast of India (Madhu et al., 2007;
Martin et al., 2008). pH values in water samples in all sampling
locations across seasons were neutral to slightly basic (7.88 ±
0.04) with no significant fluctuations. pH of a waterbody is
mainly influenced by induced anthropogenic disturbances in the
form of domestic or industrial effluents. Variations in pH were
minimal as the study locations were mainly oligotrophic to
mesotrophic. The salinity during monsoon season in central and
upper reaches (stations S1 – S4) was lower (5.6 ± 2.58 ppt)
compared to lower reaches (stations S5 and S6) with (18.1 ± 2.9
ppt). A marked wider salinity gradient was observed in all the
stations during different seasons. The upper (S1 and S2) and the
middle reaches (S3 and S4) with an influx of freshwater during
monsoon had freshwater regime. However, the salinity levels
peaked to 30 ppt with the onset of the summer season
(pre-monsoon). The salinity levels were distinctly different
across stations during post-monsoon with low salinity levels
(6.25 ± 2.47 ppt) in upper reaches, mid salinity
levels (18.12 ± 2.29 ppt) in central reaches and high salinity
(25.37 ± 1.59 ppt) in lower reaches of the estuary.
Nutrient levels across the stations were more of temporal
dilutions due to anthropogenic inputs. There was no trend in
nutrient variation across seasons among the stations and the
results were highly location specific and prone to non-point
source pollutions. Higher nitrate levels were observed (5.12 ±
0.57 µg/L) during pre-monsoon season followed by post-monsoon
(4.875 ± 1.11 µg/L) and monsoon (4.36 ± 0.58 µg/L). Phosphates
showed fluctuations within stations across different seasons
from (1.43 ± 0.09 µg/L) to (2.43 ± 0.54 µg/L). Silicate levels
showed distinct variability (Figure 4.b-d) across seasons among
stations, with higher silicate levels observed during monsoon
(6.40 ± 1.82 µg/L), followed by post-monsoon (5.04 ± 2.66 µg/L)
and pre-monsoon (av. 3.90 ± 2.34 µg/L). The variation in
silicate range was both stations as well as season-specific and
comparable to earlier studies (Madhu et al., 2007; Srinivas et
al., 2003) in the two tropical estuaries along the Indian west
coast.
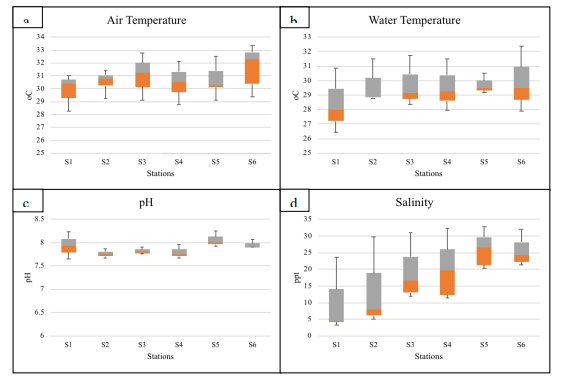
Figure 4 Variation in Physico-Chemical
Parameters across the Sampling Stations during pre-post and
monsoon Seasons (4.a-d)
Figure 5.a-d reflect variations in DO and
nutrient levels. DO was lower (6.33 ± 0.26 mg/L) during
pre-monsoon (6.33 ± 0.26 mg/L) and post-monsoon (6.72 ± 0.88
mg/L) compared to monsoonal DO (8.44 ± 0.52 mg/L).
Higher DO levels during monsoons could be attributed to the
influx of rainwater and subsequent riverine catchment run-offs
reaching the estuary. The temporal variation of DO among the
stations during monsoon was higher, evident from Figure 5.a. DO
levels observed in this region were relatively higher compared
to Cochin backwaters (Madhu et al., 2007).
Effect of hydrological parameters on Species
Richness
Two-way ANOVA analysis revealed a significant variation in
species richness with salinity, DO, and nutrients (nitrates,
phosphates, and silicates) across stations (P=0.015) and seasons
(P<0.05%).
Diatom Diversity, Dominance, and Species Composition
An estuary is a dynamic aquatic eco-system that resonates with
spatial and temporal changes. A total of 85 different species
(Appendix 1) of diatoms were recorded with similar species
occurring at different stations in one season. Station wise
overall species diversity indices (Shannon-wiener Diversity) and
Simpson’s dominance indices were estimated and are given in Fig.
6. The Shannon’s diatom diversity index (H’) was maximum for the
mesohaline, upper reach station (S2) of the estuary with H’ =
3.19 and the least diversity was observed at the polyhaline
station (S5) in the lower reaches of the estuary with H’ = 1.73,
while all other 4 stations had intermediate H’ values. Simpson’s
dominance index (D) was the highest for S1 and S6 with values D
= 0.9 and 0.86 respectively due to the outnumbering dominance of
Coscinodiscus subtilis and Navicula lanceolata
in station S1, Melosira sp. and
Navicula spp. in station S6 over other
species.
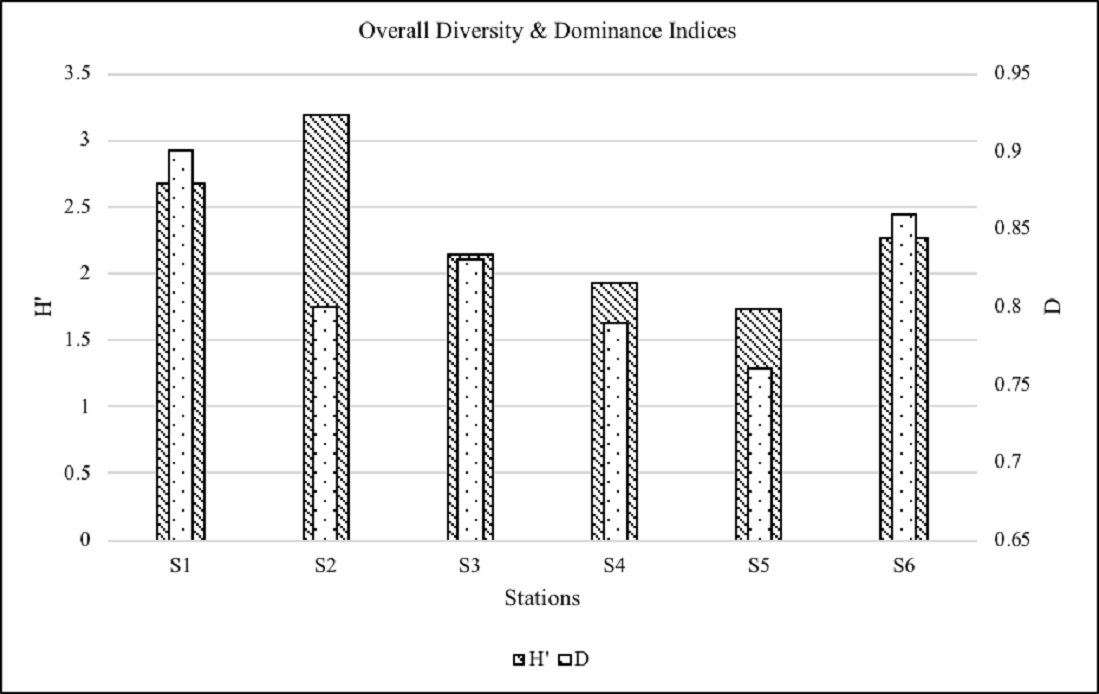
Figure 6: Diversity & Dominance Indices of
the Sampling Stations
Substrata wise Species Richness and Abundance
The data recorded substrata wise for a period of one year were
consolidated to understand the role of substrata in species
assemblage (Figure 7). The results demonstrated the highest
species abundance (36%) at S2 followed by S3 (31%). Station S6
showed the least species abundance (4%). Stations S4 and Station
S5 contributed to 11% and 5% of species abundance respectively.
Among the different substrata considered, sediments had maximum
epipelic diatom species richness of 40, 36, and 28 respectively
at stations S2, S3, and S4. Higher species richness in sediments
was followed by stones/rocks (Epilithons) at almost all the
stations. Epiphytons attached to plant substrata had the
third-highest species richness in S2, S3, and S4 stations with
species richness of 35, 21, and 23 respectively, which is
similar to the earlier report (Townsend and Gell, 2005). Lower
species richness and poor community structure are due to the
grazing pressure. Episammons (diatoms growing on sand) had the
least species richness in polyhaline lower reaches stations S5
and S6 with S = 12 and S = 18, due to persistent wave action,
washing away the community leading to lower diatom affinity in
that substrata.
Species Tolerance
The presence of a species, its abundance as well as its
distribution is largely determined by their level of tolerance
to abiotic environmental factors. Tolerance is the ability or
endurance of a species to survive in hostile environmental
conditions. When a species is exposed to prolonged unfavorable
conditions, the species tends to build up resilience to
withstand harsh environmental conditions and in turn evolve as a
tolerant species having higher ability to survive under wider
ranges of fluctuating conditions, which is observed in certain
diatom species with the consistent presence in fluctuating
environmental conditions (seasonal variability). These tolerant
species abundances w.r.t different seasons at each station is
illustrated in the form of a radar plot in Figure 8. The species
present during all the three seasons with their relative
abundances greater than 15% were considered as “tolerant
species” capable of surviving fluctuating environmental
conditions and their season-wise relative abundances were
plotted, which illustrates the site-specific abundances of
tolerant species in premonsoon, post-monsoon and monsoon. The
graph was generated by clubbing the tolerant species to the
genus level.
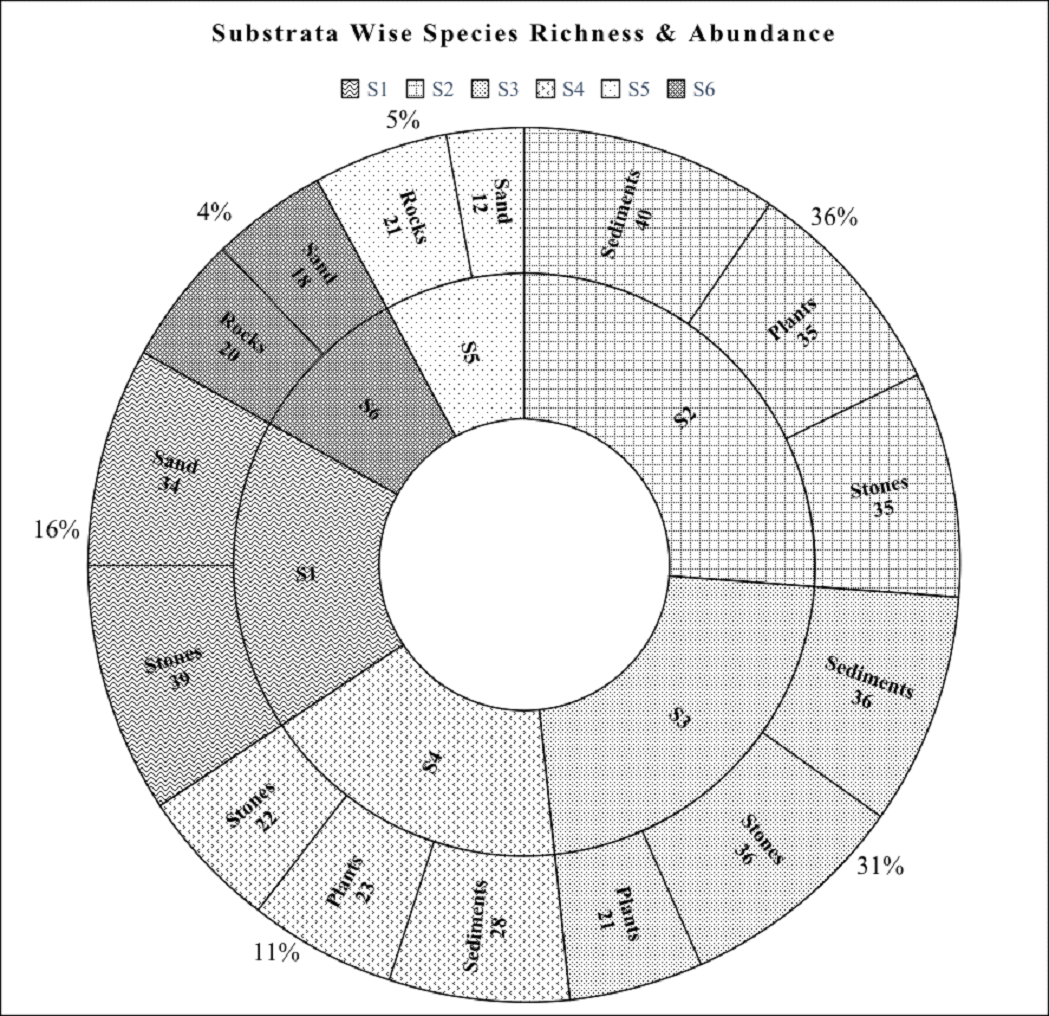
Figure 7 Pie chart representing SR and SA w.r.t
Substrata
Overall tolerant species’ abundance was highest during
pre-monsoon, followed by post-monsoon and the least abundance in
monsoon season. During premonsoon, the species abundance of Cyclotella
meneghiniana at station S2 was the highest (74.5%)
followed by consortia of Navicula sp. (Navicula
lanceolata, Navicula bicapitata, Navicula
forcipata, Navicula mutica and Navicula
amphisbaena) with 68.9% at station S3. Polyhaline (S5)
station showed a dominance of Melosira spp. with
corresponding species abundance of 72.7% during the premonsoon
season. Though the abundance of these species showed a marked
peak during pre-monsoon season of the year, the presence of
those species was recorded at other seasons as well with varying
abundance levels, proving the species to be tolerant with
evolved ecological adaptations to season-based dynamism
prevailing in real-time estuarine systems.
Season wise analyses of species’ relative abundances reveal
higher relative abundance during premonsoon with >15% of
tolerant species followed by post-monsoon and the least relative
abundances were observed during monsoon season (due to influx of
freshwater). As per the tolerance results primarily determined
by their presence in almost all the seasons with their
corresponding species-wise relative abundances to be >15%,
the diatoms belonging to the genus of Achnanthes spp.,
Nitzschia spp., Navicula spp.,
Cyclotella spp., Melosira spp. were
found to be tolerant with consistent availability at different
stations as well as during different seasons.
Among the pennate ones, the genus belonging to Amphora
and Nitzschia are known to have pronounced
heterotrophic capabilities (Linkins, 1973; Werner, 1977). Most
of the Nitzschia sp. is known to be obligate
heterotrophs with their habitat preferences in strong
heterotrophic environments like decaying piles of seaweeds, with
little or nil light penetration. Cyclotella
meneghiniana was also known to have strong
heterotrophic capabilities (Horner and Alexander, 1972; Lewin
and Hellebust, 1976; Lylis and Trainor, 1973). Another dominant
taxon observed very frequently in lower reaches (S5 and S6) was
known to be a taxon frequently encountered in higher insolation
regions, especially in tidal pools and upper inter-tidal
regions. This taxon was proven to be relatively tolerant of
inter-tidal exposure to desiccation (Castenholz, 1963; Stevenson
et al., 1996) which could have given Achnanthes sp. the
ability to survive at higher insolation over large boulders
subjected to prolonged intertidal exposure. Melosira
sp. could dominate in varying pH conditions and warmer
waters with higher light intensity. It is found that Navicula
sp. which occurs mostly over the sediment surfaces and
on other aquatic plants are facultative heterotrophs that
utilize organic matter found in the substrate (Werner, 1977).,
which justifies dominance of certain species across different
seasons in more than one station.
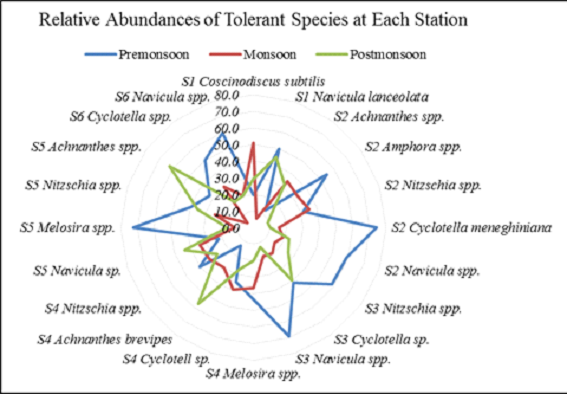
Figure 8 Relative abundance of tolerant species
w.r.t seasons
*spp. represents species composition with more than one
diatom species at respective stations from S1 to S6
S2 Achnanthes spp. – Achnanthes
brevipes, Achnanthes longipes
S2 Amphora spp. – Amphora salina, Amphora
ovalis
S2 Nitzschia spp. – Nitzschia obtusa,
Nitzschia sigma, Nitzschia apiculata, Nitzschia
fasciculata, Nitzschia panduriformis
S2 Navicula spp. – Navicula forcipata,
Navicula amphisbaena, Navicula permagna
S3 Nitzschia spp. – Nitzschia obtusa,
Nitzschia sigma, Nitzschia fasciculata, Nitzschia
acicularis, Nitzschia panduriformis
S3 Navicula spp. – Navicula lanceolata,
Navicula bicapitata, Navicula forcipata, Navicula mutica,
Navicula amphisbaena
S4 Melosira spp. – Melosira varians, Melosira
jurgensii
S4 Nitzschia spp. – Nitzschia obtusa, Nitzschia
fasciculata, Nitzschia sigma, Nitzschia acicularis
S5 Melosira spp. – Melosira lineatus,
Melosira jurgensii
S5 Nitzschia spp. – Nitzschia obtusa, Nitzschia
sigma, Nitzschia apiculata, Nitzschia fasciculata
S5 Achnanthes spp. – Achnanthes brevipes, Achnanthes
longipes
S6 Cyclotella spp. – Cyclotella meneghiniana,
Cyclotella operculata
S6 Navicula spp. – Navicula lanceolata,
Navicula granulata, Navicula mutica
S6 Cyclotella spp. includes Cyclotella
meneghiniana, Cyclotella operculata
Clustering based on Species Abundance
Month-wise species composition along with its relative abundance
of diatoms was organized season-wise and subjected to
agglomerative hierarchical clustering (Figure 9) to understand
the species predominance and season sensitivity. Cluster
analysis resulted in three distinct clusters based on their
species abundance across different seasons. Cluster 1 formed the
major group of diatom species that are sensitive and
predominantly either freshwater species or euryhaline
species.
The presence of which is observed only in specific sites at a
particular season with its relative abundance <2%. Cluster 1
includes freshwater species such as Cocconeis
placentula, Aulacoseira granulata and salinity
tolerant euryhaline species such as Amphiprora alata,
Eunotia flexuosa, Spermatogonia sp., Pleurosigma
marcum etc., with least abundance recorded in one of
the three seasons. Cluster 2 formed the second major group with
a moderate species abundance of >5%. Cluster 3 formed the
dominant groups with a higher relative species abundance of 8% -
15%. Out of 86 different species of diatoms, more than 80% of
the population were either recorded only in upstream (less
saline) regions during monsoon periods or only at downstream
regions during pre/post-monsoon seasons forming cluster 1. Those
species could be collectively termed as sensitive species, the
presence or absence of which is influenced by one or more of the
environmental parameters and even the slightest change in those
parameters could have led to the species turnover by tolerant
species. The species recorded in the upstream regions were found
to exhibit predominant freshwater habitat characteristics. The
species that exhibited such characteristics include Synedra
ulna, Aulacoseira granulata, Bacillaria
paradoxa, Cocconeis sp. Gomphonema
sp., Cymbella sp., Pinnularia sp.,
and Diploneis sp. (Appendix 1). The presence of
freshwater species was confined to upstream and middle estuarine
portions in monsoon season. On the other hand, species like Biddulphia
laevis, Licomophora sp., Amphiprora
alata, Epithema argus were found only in
downstream (S5 and S6) stations of the estuary. Hence these
species could be considered as salinity loving/euryhaline
species and their absence during monsoon and post monsoon could
be attributed to overall low salinity of the estuary during
those two seasons.
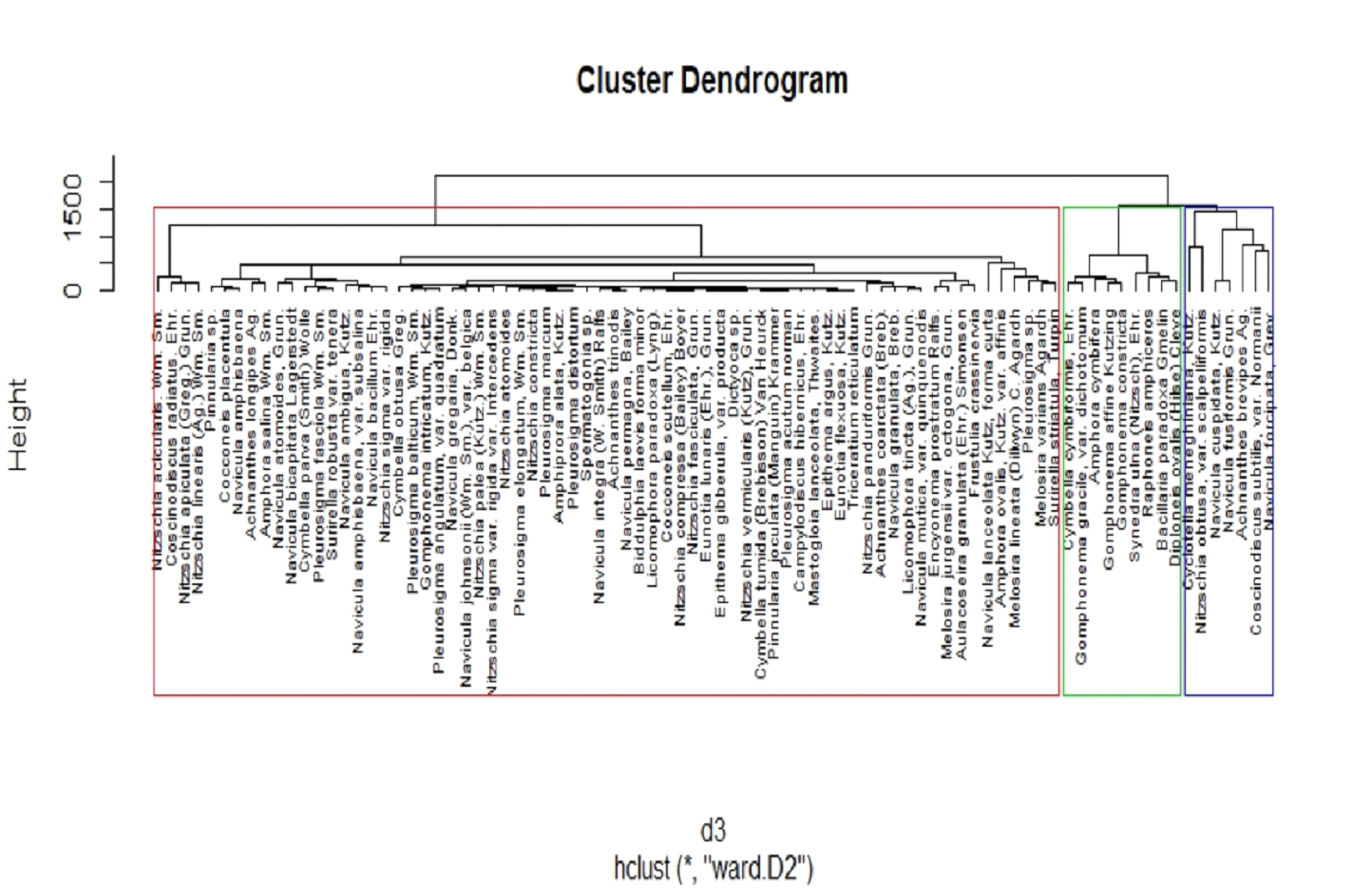
Figure 9 Agglomerative Hierarchical Clustering
of Species w.r.t Species Abundance
Season-wise Variation in Species Composition
Non-metric multidimensional scaling analysis was performed to
understand the dynamics of the diatom community structure among
stations by considering season-wise species abundance across
each station (Figure 10). NMDS is a rank-based approach in which
the ordination is achieved through an iterative process based on
pairwise dissimilarity in a low dimensional space. Here NMDS is
plotted considering species abundance summed up season-wise to
understand the commonness in species across stations. The plot
shows ordination hulls formed with respect to pre- post- and
monsoon seasons across stations (S1 PRM – S6 PRM), (S1 M – S6
M), and (S1 POM – S6 POM). The ordination hulls generated
season-wise, which demarcates tolerant species that are present
throughout the year from station-specific sensitive species. For
example, diatom species like Melosira lineatus (MLI),
Nitzschia obtusa (NIOB), Gomphonema gracile (GG),
Cyclotella meneghiniana (CM), Coscinodiscus
subtilis (CS) were present in the overlapping
intersections of all three ordination hulls indicating its
presence during all three seasons at different stations. Whereas
species like Achnanthes brevipes (AB), Achnanthes
longipes (AL), Amphora salina (AS), Navicula
amphisbaena (NAM), Melosira jurgensii (MJ)
were observed only during monsoon and pre-monsoon seasons at
more than one study locations. Whereas Pleurosigma
angulatum (PAN), Cocconeis scutellum (COS),
Navicula ambigua (NAG), Coscinodiscus radiatus
(CR) were present during pre- and post-monsoon seasons in one or
two study locations. Whereas species like Frustulia
crassinervia (FS), Aulacoseira granulata
(AUG), Licomophora paradoxa (LP), Nitzschia sigma
var. intercedens (NISV), Pleurosigma acutum
(PAC), Gomphonema intricatum (GI), Cymbella
parva (CP) were confined to one particular
station with insignificant relative abundances and observed
during only one of the three seasons.
Tolerant Species for Bioremediation and Biofuel
Recent research on third-generation biofuel production is
increasingly concentrating on using wastewater as a source of
nutrients for the growth of microalgae. If a biofuel industry is
to be set up based on open/raceway ponds using wastewater as a
source of nutrients, running the industry at consistent
productivities throughout the year proves challenging as
contamination control becomes quite difficult in such open
systems. The introduction of monoculture strains or sensitive
species further complicates the scenario as the pond is
continuously exposed to both diurnal and seasonal variations in
weather as well as water quality used for biomass enrichment.
Hence, phyco-prospecting consortia of tolerant species with
year-round availability irrespective of
microclimate/environmental conditions would be a better choice
in averting the risks of contamination or crash encountered in
monoculture sensitive strain-based cultivation systems. Thus,
from the results of the present study, it is apt to consider
tolerant diatom or consortia of tolerant diatoms capable of
exhibiting robust growth despite fluctuations and variations in
the environmental factors that greatly influence its growth. The
species having potential for phyco-prospecting evident from the
results of the present study are Amphora sp., Nitzschia
sp., Navicula sp., Achnanthes sp.,
Melosira sp., Coscinodiscus sp., and Cyclotella
sp. The above-mentioned species when chosen as a
candidate strain or as consortia of strains for
phyco-prospecting has better chances of survival in open
pond/raceway pond systems exhibiting enhanced biomass
productivities.
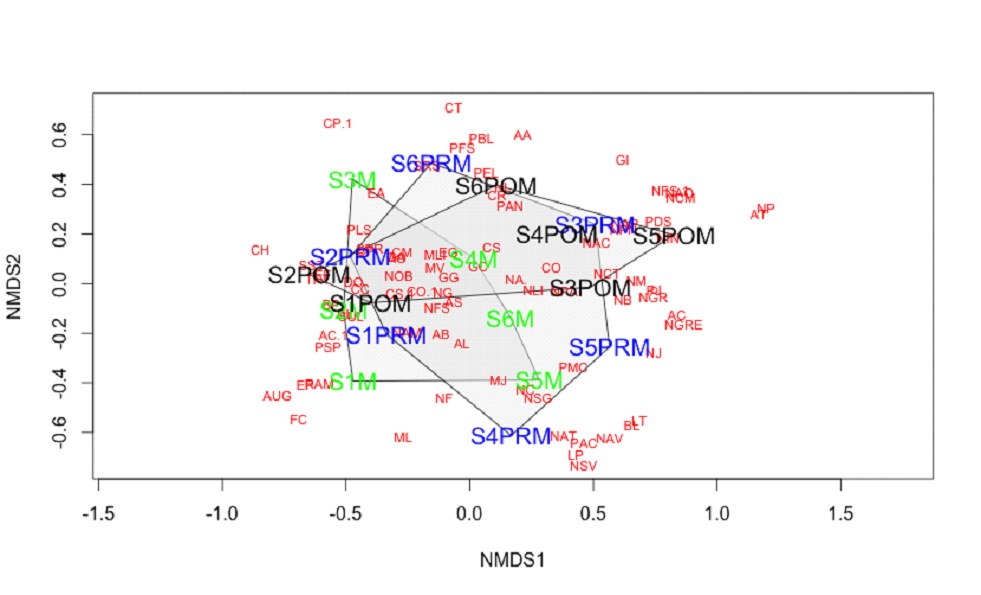
Figure 10: nMDS Plot based on Site-wise and
Season-wise Species Abundance
*The acronyms of species names used in NMDS graph are in
Appendix 1
Lipid Potential of Select Tolerant Diatom
Strains
Microalgae are widely considered as biodiesel feedstocks due to
its better lipid productivity and shorter cycling period with
quick multiplication rates when compared to conventional
terrestrial oil seeds. Many kinds of research around the globe
had focussed on lab-scale cultivation of monoculture strains to
estimate lipid productivity. Studies so far on Achnanthes
sp. under lab conditions showed a lipid content that
varies from (19.6 – 27.7) % based on dry cell weight (Zhao et
al., 2016). Lipid content studies on
Nitzschia spp. showed values ranging between
(37.5 – 46) % (Sheehan et al., 1998; Zhao et al., 2016). Melosira sp.
exhibited lipid content varying from (10.52 – 33.01) % in two
different studies (Chen et al., 2012; Renaud et al., 1994). Amphora
sp. had shown the highest lipid content reported so far
on diatoms with 49.95% (De La Peña, 2007). Different species of
Navicula, experimented under varied conditions showed
lipid content with 17.3 – 39.84% based on dry cell weight
(Fields and Kociolek, 2015). These results corroborate the
prospects of select tolerant species as potential biofuel
strains.
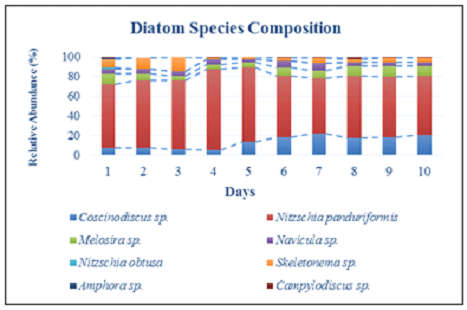
Figure 11: Laboratory Experiments on Growth Rate
and Species Composition
Laboratory Experiments to Elucidate the Lipid Potential
of Indigenous Diatom strains
Benthic diatoms were collected from rocks from one of the study
sites (S4) and immediately inoculated in F/2 media under sterile
conditions. The nutrient enrichment provided by F/2 media
facilitated a mixed consortium of diatoms to grow in the culture
with a working volume of 250 mL. The light intensity was
maintained at 90 µmol m-2 s-1 and the
temperature was maintained at 29 ± 2oC. The salinity
of the cultures was maintained at 28 psu. The nutrient-enriched
mixed cultures were then analyzed for species composition
variations throughout the growth period as well as its lipid
content once the cell reached its stationary phase. The
consortium consisted of Coscinodiscus sp., Nitzschia
panduriformis, Melosira sp.,
Navicula sp., Skeletonema sp.,
Amphora sp., Camphylodiscus sp., and Nitzschia
obtusa. Figure 11 represents the changes in the
relative abundance of each species in the consortium during the
complete growth period of ten days. After ten days, the diatoms
were harvested and subjected to lipid extraction using a
modified Folch method.
The culture consortium was further sub-cultured as aliquots under
various treatments such as nitrogen and silicon starvation to
understand the percentage increase of lipids as dry cell weights
with respect to no treatment (control) sample. Results on
varying treatments revealed that nitrogen starvation had
exhibited the highest lipid content (17 - 25% based in terms of
dry cell weight). The biomass yield was found to be 80 – 160
mg/L. The nitrogen starved algal biomass extracted lipids were
further subjected to acid catalysed transesterification to
obtain FAMEs. The FAME thus obtained were analysed using GC-MS
to understand the types of fatty acids produced by the diatom
consortia.
Figure 12 Fatty acid Methyl Ester Profiles of
harvested algal biomass
Figure 12 represents the peaks showing variations (%) in diatom
derived FAMEs. Palmitic acid methyl ester was the dominant fatty
acid recorded followed by palmitoleic acid, myristic acid, and
trans-vaccenic acid methyl ester. These results elucidate the
potential of indigenous diatom strains consortium in the
production of third-generation biofuels.
|