 |
Scope for Algal Biofuel from Wastewater |
 |
T.V. Ramachandra 1,2,3,* Durga Madhab Mahapatra 1,2
1 Energy and Wetlands Research Group, Centre for Ecological Sciences [CES],
2 Centre for Infrastructure, Transport and Urban Planning (CiSTUP), 3 Centre for Sustainable Technologies (astra),
Indian Institute of Science, Bangalore – 560012, India.
*Corresponding author: cestvr@ces.iisc.ernet.in
Abstract
Energy plays a pivotal role in the development of a region. Dwindling stock of fossil fuels coupled with the growing demand for energy to meet the burgeoning population has necessitated exploration of environmentally sound and economically viable energy alternatives. This also helps in mitigating carbon emissions. Biofuel based on microalgae are emerging as one of the most promising sources because of algae’s high photosynthetic efficiency and faster replication as compared to other energy crops. However optimisation of the conditions for the growth and technologies for biomass harvest and energy extraction are necessary for sustainability together with a cost effective way of algal cultivation. Domestic wastewaters potentially provide economic and sustainable means of dense algal growths. Algae have the ability to uptake nutrients which aid in the treatment of wastewater. The extraction of lipid from micro-algae grown in wastewater would serve the dual purpose of cost effective waste treatment and help in meeting the regional energy demand. This communication addresses the role of algae in sewage fed ponds in the treatment and the prospects for biofuel production.
Keywords: Algae, Biomass, Biofuel, Lipids, Wastewater, Methane
|
The increased demand for energy at an unprecedented rate coupled with the perishing stock of fossil based fuels and increase in Greenhouse Gases (GHG’s)1 and escalation in procurement prices has necessitated cost-effective, environmentally sound viable energy alternatives. About 90% of the global primary energy consumption is currently met through fossil based fuels2. The urban population in India is growing at about 2.3% per annum with the global urban population increasing from 13% (220 million in 1900) to 49% (3.2 billion, in 2005) and is projected to escalate to 60% (4.9 billion) by 2030 . The increase in urban population in response to the growth in urban areas is mainly due to migration. There are 48 urban agglomerations/cities having a population of more than one million in India (in 2011). Unplanned rapid urbanisation in urban agglomerations has put a severe pressure on the natural resources evident from the decline of ecosystem’s quality. Sustained inflow of sewage to the surface water bodies has contaminated and posed a threat to the surface water and the ground water resources. Enrichment of nutrients has led to the emission of GHG’s contributing to the global warming3.
Globalization and liberalization policies of the government has spurted the economic activities. With the increase in economic activities, the dependence of fossil fuel based energy sources and consequent GHG emissions have increased rapidly in recent times. Consequent to this policy change are increase in urbanization and concentrated economic activities in certain load centers resulting in higher energy consumption and also higher mobility. Emission of NOx, SOx, CO from petroleum derived fuels have enhanced the GHG levels in the atmosphere contributing global warming and consequent changes in the climate4. This has necessitated the adoption of clean and green renewable technologies, which helps in meeting the energy demand and also in sequestering the atmospheric CO2. Minimised dependency on fossil reserves and usage of renewable and carbon neutral fuels foster the environmental and economic sustainability4,5. The demand for primary energy sources has been increasing during the last decade and is expected to rise by 40% during the next two decades6,7.
As alternatives the crop-based biofuel albeit being economically competitive compared to fossil fuels have failed because of inconsistencies in production and lower energy returns. Use of crops for biofuel generation competes with the food crops and possesses a threat to food security5,8. Microalgae are emerging as one of the most promising sources of biofuel because of their high photosynthetic efficiency, mitigation of GHGs4 and faster replication as compared to any other energy crops. Although, the concept of using microalgal lipid as a source of fuel is very mature, its approach in benefiting both environmental and energy-related is a frontier research area today. Algal community for the production of lipid depends on the physical, chemical as well as biological variables of aquatic ecosystems. Algae based biofuel technologies help as renewable sources of energy and are C neutral9. The biofuel prospects of algae grown in sewage fed ponds have been explored. Algae also aid in the uptake of nutrients and hence in treatment of wastewater10.
Algal biofuels have proved to be the potential alternative as it does not impact the agriculture and have the abilities to grow profusely in extreme environments with higher productivities11. Algal biofuels as carbon neutral agents can play a vital role in meeting the energy targets to replace fossil based transportation fuels12. The efforts in recent times reveal that liquid fuels used in transport sectors can be replaced by the C neutral algal biodiesel4. In recent years, there has been spurt in the usage of liquid biofuel in the transport 6. The transport sector in India consumes about 16.9% (36.5 mtoe: million tonnes of oil equivalent) of total energy (217 mtoe in 2005-06). Various energy sources used in this sector are coal, diesel, petroleum (gasoline) and electricity. Road, rail and air are responsible for emission of 80%, 13% and 6% respectively. Vehicular emissions account for about 60% of the GHG’s from various activities in India13.
|
The hydrocarbon based fuels have played a prominent role in developing nation’s economy. The current rate of fossil oil utilisation reveals an increasing trend in consumption to meet the growing demand in the energy and transport sectors. Indian oil reserves are scanty and the country imports major portion to meet the demand. India being the fourth largest consumer of oil in the world consumes about 352 million litres/day. As per the reports from Ministry of Petroleum and Natural Gas, Government of India 2010, the total amount of oil production in India is less than 2,50,000 tonnes per year compared to India total reserves of 775 million metric tonnes (MMT) of crude oil. India’s major oil fields are gradually showing the declining trend and some locations are already exhausted and hence the Nation imports more than 80% of consumed oil which accounts to 114 million tonnes/year (Figure 1). Oil and gas as fuels contributes about 45% of the total energy needs14 and the distribution of energy supply is depicted in Figure 2.
The demand for oil is likely to go much higher and expected to reach 250 M tonnes by 202415. The report from EIA16 shows annual oil consumption growth reaching nearly 100,000 barrels/day (11,735,000 lt/d). A look at the fuel use in terms of oil consumption shows that from 2000 to 2010, crude oil consumption in India has increased by more than 80% (Figure 1) with an average annual growth rate of 5%. The planning commission of India has brought out an extensive report on the prospects of biofuels in India. The National Biodiesel Commission has advocated blending of 20% biodiesel and 10% ethanol with petro-diesel during 2011–201218. About 13.4 Million Tonnes of biodiesel were targeted to achieve the national mission of 20% blending for the current year. Figures 3A) and 3B) elucidates the various Energy sources and Sectoral energy distribution for the year 2006.
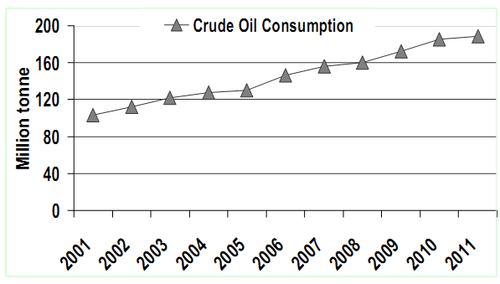
Figure 1: Crude oil consumption in India
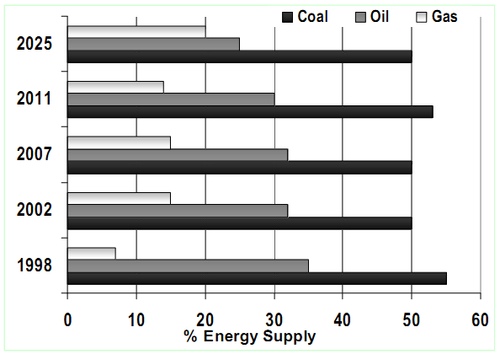
Figure 2. Distribution of energy supply in India
(Modified from Technical Note on Energy,
Planning Commission, Govt. of India, 1998–1999)
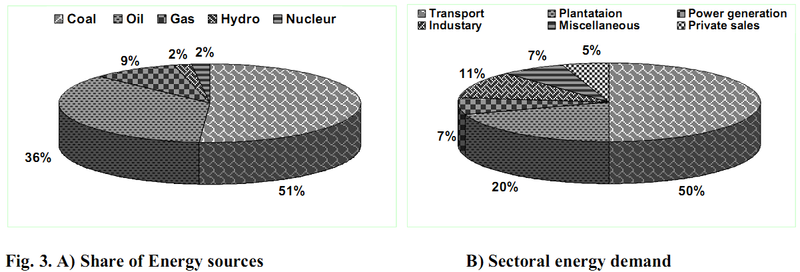
|
Algae have the potential to generate significant quantities of biomass and oil suitable for conversion to biodiesel. The algal species have higher growth rates in natural conditions and higher biomass productivities compared to oil-crops in terms of land area requirement and are economic that reduces GHG’s as replacement of fossil fuels by lipid production19. The lipids (TAG’s principally) can be extracted and purified from algae and then transesterified to biodiesel20. Biodiesel generation from algae has attained significant interesting recent times. Several studies have revealed a much higher potential of oil from algae compared to oil seeds21. A lot of research focus has been given to screening oil rich algae and finding suitable growth conditions for greater oil yields22. Many studies for scrutinising bio-chemical environments that triggers neutral lipid accumulation (TAG’s) in algal cells for example nutrient stress as N and P limitation23 and Fe limitation24 etc. have been undertaken. One of the major limitations of such type of approaches is decreased biomass productivities of cells. So, even at higher lipid yield the lipid productivities are low22. Therefore it is suggested to have higher biomass yield than accumulating lipid in algal cells. Higher biomass yield can pave ways an economic route of energy generation through other biofuel by using different bio-chemical processes.
|
Recent estimates of Central Pollution Control Board (CPCB) indicate of 40 billion litres of wastewater generation in the country mostly from urban areas25 and about 20-30 % of the generated wastewater is treated. In most of the urbanised areas the wastewater is inadequately treated and thence has become the reason for eutrophication and increased bacterial infection resulting in deteriorating health and hygiene because of the contamination of surface and ground water resources. The average daily per capita water supply in India is about 88.73 litres and sewage accounts to 137.82 litres. Accounting for the losses ~80 lt per capita is disposed into receiving waters. The statistics of the wastewater generation to the installed treatment capacities for various cities is listed in Table .1.
Table 1: Wastewater generation in cities of India
|
City Types |
Treatment aspects |
Metropolitan cities |
Class-I cities |
Class-II cities |
Cities at Ganga Basin |
Population size |
>10 Lakhs |
>1 Lakh |
10,000 to 1 lakh |
20 million |
No. of Cities |
35 |
498 |
410 |
113 |
Wastewater generated (MLD) |
15644 |
35558.12 |
2696.2 |
2637.7 |
Treatment plant installed (MLD) |
8040 |
11553.68 |
233.7 |
1174.4 |
Percentage Treatment |
50% |
32% |
10% |
44.20% |
|
One of the most prominent advantages of algae is their adaptability to grow in polluted environments. They naturally grow at higher cell densities in nutrient rich waters and therefore can be grown in conditions with minimal freshwater requirement compared other land-based biofuel crops. Wastewaters are potential options for algal growth and subsequent derivation of algal-products as biofuels. Microalgae can be potentially utilised for low-cost and environmentally friendly wastewater treatment compared to other more commonly used treatment processes26,27.
The wastewater generated often is concentrated with high proportions of nutrients (CNP) and other mineral ions. In the developing countries there is a growing consensus on the enormity of wastewater generation and at the same time the technologies available to treat the same becomes highly expensive with chemical based systems10,11. The N and P concentrations of municipal wastewaters in the urban areas of India like Bangalore ranges from 90-30 and 10-33 ppm respectively10. Algae being the most rapid assimilators of nutrients effectively grow in these nutrient rich environment makes them the most suitable candidate for low-cost wastewater treatment28. Efforts show energy generation from algal biofuel with environmental and economic sustainability4,19,21, 27.
India has enormous wastewater generated everyday (~40 billion litres/d) and an average solar insolation of 5 kWh/m2/day. Wastewater with abundant nutrients (particularly C, N and P) poses a suitable environment for the profuse growth of algae. Algae grow to their peak productivities at an insolation of 5-6 kWh/m2/day with the higher photosynthetic efficiencies. Regular harvesting of algae grown in wastewater for biofuel production will provide the sustainability of energy sources while treating wastewater. Field experiments reveal the potential of wastewater grown algae in tropical climates and to produce cost-effective biofuel while treating wastewater. This would ensure water, energy and health security.
|
Nature and the degree of variability of the wastewaters generated are very high. Even in such wastewaters different types of algal communities thrive by their ability to utilise abundant organic carbon and inorganic N and P in the wastewater. The use of algae in nutrient removal in wastewaters is not new and has been known from 1950’s29 in the form of wastewater stabilisation (oxidation) ponds or raceway algal ponds with mechanical mixing30.
A major requirement of wastewater treatment in the rapidly urbanising regions is the removal of high concentrations of nutrients in particular, N and P, and to some extent C. Most of the C released into the sewer system is degraded anaerobically and subsequently aerobically during its flow regime in open sewers and storm water drains31,32. The existing treatment units could partially treat the wastewaters up to secondary levels10. However the limiting nutrients as N and P are difficult to remove from wastewater in particular. Most of the N present in the system is in the form of Amm-N and Nitrate-N that can be potentially up-taken and immobilised in algae10. Algae are efficient in removing N, P from wastewater33 and thence are key players for nutrient remediation particularly during the secondary and tertiary treatment phase of wastewater.
P removal efficiencies in algae-based treatment systems is relatively high compared to chemical treatment30. Figure 4 depicts the stocking of polyphosphates and calcium phosphates adsorbed over the dried algal solids. SEM image shows a dried algal cell having a mean diameter of ~45 µm. The algal based wastewater technologies are advantageous over conventional ones as they are simple, cost effective low energy intensive, thus best suiting the developing countries. Significantly higher rates of oxygen generation from photosynthetic algae (6-28 ppm) offset the requirement of mechanical aeration systems which has a high initial and operational costs34. Oxygen in the dissolved forms (in treatment units) enhances the rate of heterotrophic bacteria to remove organics and other ionic substances and treat the wastewater35. Furthermore the algal treatment systems enhances the sustainability of the treatment systems by avoiding the generation of bi-products and additional pollutants like sludge thereby closing the loop for efficient nutrient recycling. The biomass in these ponds are rich in nutrients that can directly applied to land as low-cost fertilisers, as animal feed35 or can be made to use in many different ways that satisfies our biofuel needs.
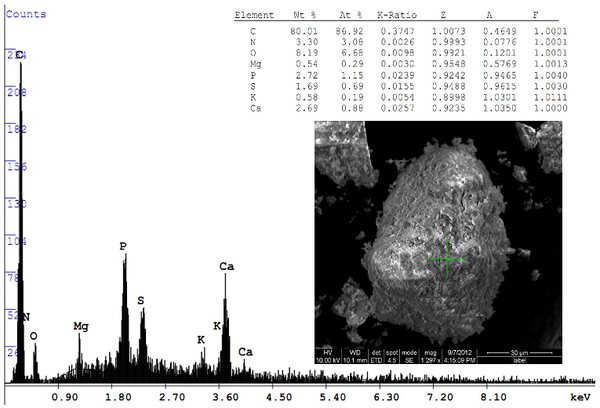
Figure 4: SEM image elucidtes Polyphosphate accumulation in algal solids
There have been significantly higher numbers of studies on growth of algae under a wide variety of wastewater environments at different conditions. Most of these studies have focussed on urban wastewaters as municipal sewage. These studies have primarily looked at evaluating the potential of algae for removing N and P, and in some instances metals and other minerals from wastewater. Many studies have focussed on factors that are responsible for maximum algal biomass production and mechanisms of algal biomass harvest from wastewaters. This study provides a lot of insights for the evaluation of wastewater algae as potential biofuel options.
|
Algal growth and development depends on a multitude of variables such as physicochemical variables (pH, growth medium temperature, light availability, gas exchange systems, etc.), nutrient concentrations (primarily C, N and P) and other ions, and efficiencies. Studies on the factors affecting growth reveal that algal growth significantly increased at longer photoperiods and addition of CO2 and in the contrary increased temperature regimes during growth substantially decreased the density of algal biomass36.
The urban wastewaters have higher concentrations of nutrients - C, N and P. The major fraction of N is in the form of ammoniacal–N i.e. 35 ppm10 which sustains many algal species mostly dominated by Chlorophyceaen members comprising Chlorella sp., Monoraphidium sp. and Chlorococcum sp. Contrary to this there are many studies that highlights the lethal effects of higher Amm-N on algae due to ammonia toxicity36-38. The algal communities in a sewage fed urban lake (at Bangalore) were responsible for about 76% N removal and 60% C removal10. Similar findings were also found in earlier studies where chlorophytic algae have been shown to be particularly tolerant to many wastewater conditions and are very efficient at accumulating nutrients from wastewater39. Studies also reveal the presence of euglenophytes in wastewaters with higher organic loading which derives nutrients from the mixotropic environments. Chlorella sp. and Scenedesmus sp. are usually predominant of the phytoplanktonic communities in oxidation ponds40 and in high-rate algal ponds9.
|
Euglena sp. has been recently characterised in wastewater treatment ponds in India (Bangalore and Mysore, Karnataka). They have a higher biomass productivities in raw sewage and grow heterotrophically in dark and have a mixotrophic mode of nutrition on variable C and N (NH4-N) with growth rates of 155 mg/l (unpublished data) compared to 73 mg/l under phototrophic conditions for growing Chlorella minutissima in 10 days41. These experiments prove euglenophycean members as better nutrient assimilators in raw wastewater systems. Algal growth and nutrient removal studies have also been conducted on artificial wastewater42,43, which lack solid organic matter and does not mimic the original wastewater conditions. The growth of Chlorella and Scenedesmus has been studied widely as these species grows well in sewage effluents44. These species show a very high (>80%) nutrient removal efficiency and in many instances results in almost complete removal of ammonia, nitrate and total P from secondary treated wastewater39, indicating the potential of microalgae for tertiary sewage treatment.
|
The capability of algal communities to thrive under acute wastewater signifies the potential of these nutrient rich resources as suitable sustainable natural growth medium for biofuel feedstock. These algal communities can stock significant amount of lipids in the cell. The nature and type of lipids (saturates, unsaturates, polyunsaturates and glycol/phosphor lipids or TAGS) and the quantity of lipids produced (max-80% of the cell dry weight) depends on the nature of species and the algal growth conditions4,22.
The unlimited nutrient supply in wastewaters would result is high algal cell densities and higher lipid productivities. Several laboratory based studies focussed in biofuel from wastewater have been carried out in batch cultures, semi-continuous cultures and in bioreactors with ponds have reported satisfactory lipid accumulation in wastewater grown algae yielding low (<15%), moderate (25-30%) lipid content and in many cases a very high lipid yield is achieved.
The present studies undertaken to screen the algae with highest lipid content showed Chlorococcum sp. which had the highest lipid content (~28 %) followed by Euglena sp. (~24%), Phormidium sp. (~18%) and Microcystis sp. (~8%). Algae mostly grown on municipal wastewater and the biomass of the cells ranged from 20–35 mg/l/d and the wastewater produced each year desired to be used for the entire year would yield the algal biomass of 16-28 tons/ha/yr to produce an estimated lipid yield of 3260-3830 L/ha/yr28. The batch culture growth studies in municipal wastewaters of Chlamydomonas reinhardtii revealed higher growth rates (100% centrate wastewater) with a lipid content of ~17% on a dry wt. basis 45. The same cultures being transferred to bio-coil showed a consistent growth in wastewaters with increased lipid content of 26% on a dry wt. basis and biomass productivity of 2 g/l/d and an estimated lipid yield of 0.505 g/l/d coupled with adequate nutrient removal45. The lipid content from mixed algae cultures, isolated from sewage treatment ponds, grown in anaerobically digested dairy manure wastewater in outdoor batch cultures showed an leap in lipid accumulation In a week (14% to 29%) with an estimated lipid productivity of 2.8 g/m2/d27.
|
The transitions in the biochemical compositions were identified using FT-IR spectroscopy and the band assignments following Dean et al (2010)23 and observed the cells at wave numbers (2 cm-1 resolution and 64 scans) between 1800 and 800 cm-1. The periodically harvested and dried algal biomass showed peaks at ~1,740 cm-1 due to C=O stretching in Lipids (esters of fatty acids); ~1,650 cm-1 C=O stretching in Proteins (Amide I); ~1,540 cm-1 N-H Amides of proteins (Amide II); ~1,398 cm-1 CH3 and CH2 of Proteins (Amide III); ~1,240 cm-1 P=O Phospholipids, DNA and RNA; 1,200-1,160 cm-1 C-O-C in Carbohydrates and Polysaccharides and ~830 cm-1 C=N (ring) in nucleotides and chlorophyll pigments. The Chlorococcum sp. grown in wastewater showed higher lipid content at the end of the batch cycles due to nutrient deprivation as can be observed in the shifts of the biochemical pools at the initial and the later stages of the cycle. The interconversions of the metabolites (protein pool to polysaccharides in carbohydrates to lipids pool) can be clearly observed from the FTIR spectra.
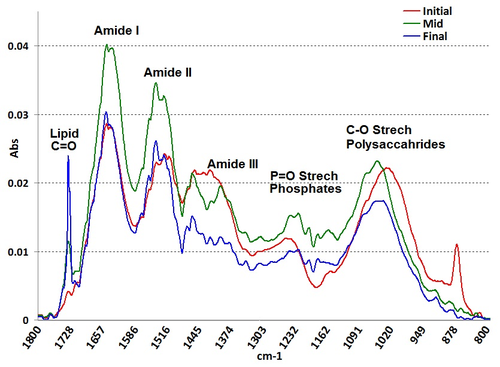
Fig. 5 FTIR bands for the compositional analysis of algae during its cultivation
*The red, green and blue curves indicates the spectra of the algal cells during the initial, middle and final stages of the cultivation
The algae (chlorophycea) isolated from the wastewaters from Varthur lake were made to grow in outdoor cultures in open tub reactors (bench scale, batch reactors 10 litres volume) having a depth of 20 cm that was fed directly with municipal wastewaters that had an average biochemical oxygen demand (BOD) concentration of 220 mg/l. The daily growth of the algal species were monitored and lipid measurements showed a hype in lipid content during the 9th day of the culture experiment just at the later stages of the exponential growth and was confirmed with Fourier transform infrared spectroscopic (FTIR) studies that showed a very prominent band at ~1740 cm-1 (lipid) in the Vibrational spectra (Figure 5). Ultrasonication was employed for cell disruption and the lipid extraction was carried following the methods of Bligh and Dier (1959)46 and the total lipid content during the experiment varied from 24.5-28.4% of dry algal biomass. The mass productivity was observed to be 133 mg/l/d from the open tubs with an area productivity of ~10 g/m2/d. The nutrient removal experiment showed 95 % of TN removal and 88% of phosphate removal.
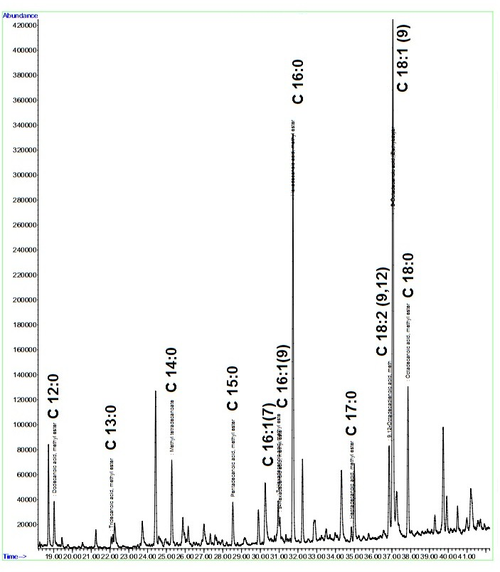
Figure 6: Chromatogram of the FAME present in Chlorococcum species.
*The peaks indicating the abundance (concentration) of the fatty acids
The gas chromatography and mass spectrometry (GC-MS) analysis of the fatty acid methyl esters (FAME) showed a higher content of important fatty acids having desirable biofuel properties47 with major contribution by the unsaturated fatty acid (UFA) oleic acid (C18:1; 42%) followed by palmitic acid (C16:0; 27%) followed by stearic acid (C18:0; 11%) and linoleic acid (C18:2; 6%) comprising of 86% of the total fatty acid constituents (Figure 6). These algal species auto-flocculated at the top of the cultures at the end of the batch cycles and thus making the harvesting easier and faster for algal biomass and oil quantification.
|
The use of algae grown profusely in the wastewater generated daily would offset the nutrient and CO2 demands. Sewage fed urban lakes is rich in nutrients with a very high and healthy aerobic bacterial microflora that generates ample CO2 through respiration. This CO2 is utilised by the micro-algae10 during photosynthesis35. The wastewater systems provide a year round availability of nutrients with a rich natural microflora for a synergistic growth of algal-bacterial systems. Constructed lagoon system removes the burden of land area requirement to grow algae for biofuel requirements. Bangalore city generates nearly 1200 MLD of wastewater daily. Belandur-Varthur lake systems receive ~500 MLD and adequate treatment of wastewater has been happening due to the natural algal growth. However, algal growth reaches optimum in the absence of macrophytes cover48. With an average efficiency of 50% nutrient removal such systems can be optimised and made sustainable by careful feed rate management and optimal retention time. The average algal cell densities found in these systems are of the order of 105-106 cells/ml. That gives an biomass productivities of ~10g/m2/d. The nutrient loads at the entry (inlet) of Bellandur lake (at upstream of Varthur)were calculated as 90-120 mg/l TOC; 75-90 mg/l TN and 20-40 mg/l of TP,.
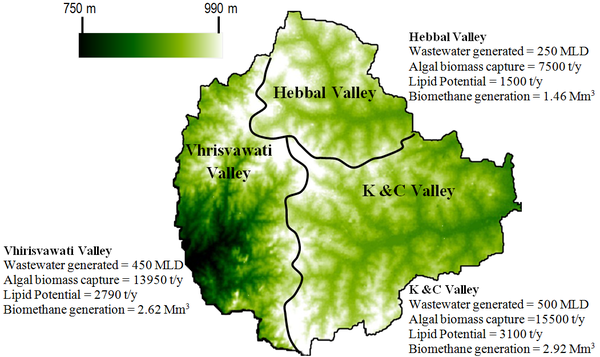
Figure 7: Algal lipid potential of Bangalore
The average annual influx of nutrients amounts to 20,000 t TOC /yr, 14,600 tTN/ yr and 5,475 tTP/yr. Number of algal species thrive under such enormous nutrient loads in the sewage fed lake. The annual biomass generation for Bellandur lake (365 hectares) is roughly 13,000 t. Out of these if 40% in harvestable and extraction process, then it still yields ~5000 t algal biomass/yr. Now taking the average lipid content of 20% (1000 t) of lipid can be extracted from this water body per annum. Similarly 640 t algal lipid/yr can be extracted from Varthur lake, the next lake in the cascading lake series. For the entire wastewater generated in Bangalore the net lipid potential corresponds to 7,200 t/yr. The spent algal-biomass after lipid extraction is ~18000 tonnes/yr and can be anaerobically digested that would yield 7 Mm3 of methane. Figure 7 depicts the lipid and bio-methane generation potential of the entire Bangalore wastewater from the three valleys. Deployment of appropriate technologies for algal biofuel would provide an additional energy security from the spent biomass. The slurry left after the anaerobic digestion can be used as an excellent nutrient media for further algal cultivation or can be used as manure (rich with nutrients) / fertiliser, which would help in stabilising our agrarian economy. In this complete loop there is no net generation of waste at the same time there is a lot of positive net energy gain that indicates sustainability. Further investigations on such type of naturally managed system and its process understanding will eventually lead to optimal wastewater treatment with efficient biofuel production. This work illustrates sustainable biodiesel production through lipid extraction from algal biomass (grown in wastewater) and is the most promising technically feasible and economically viable option. Hybrid systems of methane from the remaining residual algal biomass49 and biofuel production would boost the regional economy. A process flow diagram of the sustainable wastewater treatment with biofuel and nutrient recovery is given in Figure 8.
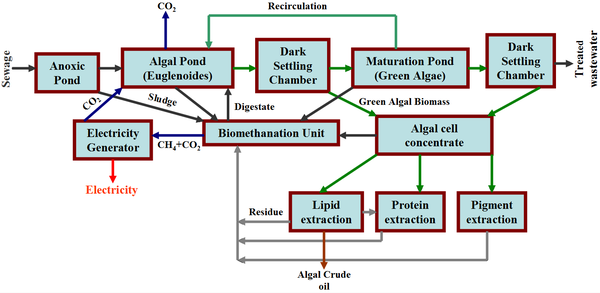
Figure 8: Sustainable algal biofuel coupled with other value added by-products
The enormous and consistent nutrient supply in sewage fed ponds would result in higher biomass productivities and higher lipid yields. Earlier studies in wastewaters conform of higher biomass yield45. However further research is required to demonstrate the technical feasibility of algal biofuel production from sewage fed ponds.
|
Algal biofuels would ensure the regional energy security as well as help in mitigating GHG’s. Sustained flow of untreated sewage into urban water-bodies have contaminated surface water as well as ground water sources evident from higher nitrate levels in the ground water sources in the vicinity of sewage fed urban lakes in Bangalore. Bangalore city generates nearly 1200 MLD of wastewater daily, and is fed urban water bodies. The average annual influx of nutrients amounts to 20,000 t TOC /yr, 14,600 tTN/ yr and 5,475 tTP/yr. Belandur-Varthur lake series receives about 500 MLD of sewage everyday. The annual biomass generation for Bellandur lake is roughly 13,000 t. Out of these if 40% in harvestable and extraction process, then it still yields ~5000 t algal biomass/yr. Now taking the average lipid content of 20% (1000 t) of lipid can be extracted from this water body per annum. Similarly 640 t algal lipid/yr can be extracted from Varthur lake, the next lake in the cascading lake series. For the entire wastewater generated in Bangalore the net lipid potential corresponds to 7,200 t/yr. The spent algal-biomass after lipid extraction is ~18000 tonnes/yr and can be anaerobically digested that would yield 7 Mm3 of methane.
The algal species were found to have higher biomass growth of >1 g/l during their exponential stages. Together with that they substantially accumulate lipoids in their cells during the end of the exponential phases a evidenced by the vibrational spectra obtained from FTIR analysis. Among the selected algae the highest growth rate was observed in Chlorococcum sp. that reached the stationary phase at the end of 9th day. The higher total lipid content was observed in Chlorococcum sp. (28 %) that grows in nutrient rich wastewater ponds and lagoons. Palmitate (C 16:0) was found out to be the most dominant among the FAME analysed. FAME analysis elucidated higher percentages of important fatty acids in the selected algal species that are necessary from biofuel combustion perspectives. With higher lipid content, a very high growth rate and having desirable fatty acids content wastewater grown algae foundin ponds and lagoons are excellent substrates for biofuel /lipid production and can be used as a cost effective option for sustainable biofuel production. The analysis demonstrates the potential of algal biofuel in meeting the energy demand. This renewable biofuel supplement the conventional fuel being used in transport and energy sectors and reduce the burden of oil imports while fostering sustainability and environmentally sound growth of a region.
|
We are grateful to NRDMS Division, the Ministry of Science and Technology, Governmant of India, Indian Institute of Science and the Ministry of Environment and Forests, Government of India for the financial and infrastructure support. We thank Shubhadip Chakraborty and Prof. P. K. Das from IPC Department, IISc for their help in ATR-FTIR Measurements. We acknowledge IISc Nano Initiative (INI, IISc) and the Central facilities, at IISc Biochemistry department for permitting to use Electron Microscopy and GC-MS.
|
- Hill J., Nelson E., Tilman D., Polasky S., Tiffany D., 2006 Environmental, economic, and energetic costs and benefits of biodiesel and ethanol biofuels. Proc. Natl. Acad. Sci. USA; 103, 11206–11210.
- Brennan L., Owende P., 2010 Biofuels from microalgae – a review of technologies for production, processing, and extractions of biofuels and co-products. Renewable and Sustainable Energy Reviews; 14: 557–577.
- IPCC. 2007. “Climate Change 2007: The Physical Science Basis. In Contribution of Working Group I to the Fourth Assessment Report of the International Panel on Climate Changes, edited by S. Solomon, D. Qin, M. Manning, M. Mariquis, K. Averyt, M.M.B. Tignor, H. LeRoy Miller, Jr, and Z. Chen, 95–103. NewYork: Cambridge University Press.
- Ramachandra T.V., Mahapatra D.M., Karthick B., Gordon R., 2009 Milking diatoms for sustainable energy: biochemical engineering vs gasoline secreting diatom solar panels. Industrial and Engineering Chemistry Research; 48: 8769–8788.
- Singh A., Nigam P.S., Murphy J.D., 2011 Renewable fuels from algae: an answer to debatable land based fuels’, Bioresou Technol. 102: 10–16.
- International Energy Agency (IEA) (2007) World energy outlook. IEA, Paris
- International Energy Agency (IEA), 2009.. http://www.iea.org (Accessed 26 August 2012)
- Khan S.A., Rashmi-Hussain M.Z., Prasad S., Banerjee U.C., 2009 Prospects of biodiesel production from microalgae in India. Renewable and Sustainable Energy Reviews; 13: 2361–2372.
- Demirbas A., 2009 Biofuels securing the planet’s future energy needs. Energy Conversion and Management; 50: 2239–2249.
- Mahapatra D.M., Chanakya H.N., Ramachandra T.V., 2011a Assessment of Treatment capabilities of Varthur Lake, Bangalore. International Journal for Environment, Technology and Management; 14: 84–102.
- Chanakya H.N., Mahapatra D.M., Sarada R., Chauhan V.S., Abitha R., 2012 Sustainability of large-scale algal biofuel production in India. Journal of Indian Institute of Science; 92:63–9.
- Yuan J.S., Tiller K,H., Al-Ahmad H., Stewart N.R., Stewart C.N., 2008 Plants to power: bioenergy to fuel the future. Trends Plant Science; 13: 421–429.
- Ramachandra, T.V., Shwetmala, Emissions from India’s transport sector: Statewise synthesis, Atmospheric Environment (2009), doi:10.1016/j.atmosenv.2009.07.015
- Ministry of Petroleum and Natural Gas, Government of India. 2010. Background material for economic editors Conference.
- Planning commission report, Government of India, 1998.
http://planningcommission.nic.in/plans/planrel/fiveyr/10th/volume2/v2_ch5_3.pdf
- U.S. Energy Information Administration (EIA) 2011. “International Energy Statistics database (as of March 2011), Projections: EIA.” Annual Energy Outlook 2011, DOE/EIA- 0383(2011) (Washington, DC, April 2011). Accessed 26 August 2011. http://eia.gov/ies
- Planning Commission, Government of India. 2003. Information from Various Ministries and the Planning Commission of the Government of India. Accessed 18 August 2012. http://planningcommission.nic.in
- Government of India, Ministry of New & Renewable Energy. 2008. National Policy on Biofuels. Accessed 19 August 2012. http://mnre.gov.in/policy/biofuel-policy.pdf
- Stephens E., Ross I.L., King Z., Mussgnug J.H., Kruse O., Posten C., Borowitzka M.A., Hankamer B., 2010 An economic and technical evaluation of microalgal biofuels. Nature Biotechnology; 28: 126–128.
- Hu Q., Sommerfeld M., Jarvis E., Ghirardi M., Posewitz M., Seibert M., Darzins A., 2008 Microalgal triacylglycerols as feedstocks for biofuel production: perspectives and advances. Plant Journal; 54: 621–639.
- Chisti Y., 2007 Biodiesel from microalgae. Biotechnology Advances; 25: 294–306.
- Griffiths M.J., Harrison S.T.L., 2009 Lipid productivity as a key characteristic for choosing algal species for biodiesel production. Journal of Applied Phycology; 21: 493–507.
- Dean A.P., Sigee D.C., Estrada, B.J.K., 2010 Using FTIR spectroscopy for rapid determination of lipid accumulation in response to nitrogen limitation in freshwater microalgae. Bioresource Technology; 101: 4499–4507.
- Liu Z., Wang G., Zhou B., 2008 Effect of iron on growth and lipid accumulation in Chlorella vulgaris. Bioresource Technology; 99: 4717–4722.
- Central Pollution Control Board. (CPCB). ‘Status of wastewater generation and treatment in India’2011. Resour. document. Accessed 18 August 2012. www.umweltbundesamt.at/.../3bStatus_of_Wastewater_India.pdf
- Pittman J.K., Dean A.P., Osundeku O., 2011 The Potential of Sustainable Algal biofuel production usingwastewater resources. Bioresource Technology; 102:17–25.
- Woertz I., Feffer A., Lundquist T., Nelson Y., 2009 Algae grown on dairy and municipal wastewater for simultaneous nutrient removal and lipid production for biofuel feedstock. Journal of Environmental Engineering; 135: 1115–1122.
- Chinnasamy S., Bhatnagar A., Hunt R.W., Das K.C., 2010 Microalgae cultivation in a wastewater dominated by carpet mill effluents for biofuel applications. Bioresourse Technology; 101: 3097–3105.
- Oswald W.J., Gotaas H.B., Golueke C.G., Kellen W.R., 1957 Algae in waste treatment. Sewage Ind. Wastes; 29: 437–455.
- Hoffmann J.P., 1998 Wastewater treatment with suspended and non-suspended algae. Journal of Phycology. 34: 757–763.
- Mahapatra D.M., Chanakya H.N., Ramachandra T.V., 2011b C:N ratio of Sediments in a sewage fed Urban Lake. International Journal of Geology; 5: 86–92.
- Mahapatra D.M., Chanakya H.N., Ramachandra T.V., 2011c Role of macrophytes in urban sewage fed lakes. Institute of Integrative Omics and Applied Biotechnology; 2: 1–9.
- Ahluwalia S.S., Goyal D., 2007 Microbial and plant derived biomass for removal of heavy metals from wastewater. Bioresource Technology; 98: 2243–2257.
- Mallick N., 2002 Biotechnological potential of immobilized algae for wastewater N, P and metal removal: a review. Bio-Metals; 15: 377–390.
- Munoz R., Guieysse B., 2006 Algal–bacterial processes for the treatment of hazardous contaminants: a review. Water Research; 40: 2799–2815.
- Ip S.Y., Bridger J.S., Chin C.T., Martin,W.R.B., Raper W.G.C., 1982 Algal growth in primary settled sewage – the effects of five key variables. Water Research; 16: 621–632.
- Konig A., Pearson H.W., Silva S.A., 1987 Ammonia toxicity to algal growth in waste stabilization ponds. Water Science and Technology; 19: 115–122.
- Wrigley T.J., Toerien D.F., 1990 Limnological aspects of small sewage ponds. Water Research; 24: 83–90.
- Ruiz-Marin A., Mendoza-Espinosa L.G., Stephenson T., 2010 Growth and nutrient removal in free and immobilized green algae in batch and semi-continuous cultures treating real wastewater. Bioresource Technology; 101: 58–64.
- Masseret E., Amblard C., Bourdier G., Sargos D., 2000 Effects of a waste stabilization lagoon discharge on bacterial and phytoplanktonic communities of a stream. Water Environment Research; 72: 285–294.
- Bhatnagar A., Bhatnagar M., Chinnasamy S., Das, K., 2010 Chlorella minutissima – a promising fuel alga for cultivation in municipal wastewaters. Applid Biochemistry and Biotechnology; 161: 523–536.
- Wang L., Min M., Li Y., Chen P., Chen Y., Liu Y., Wang Y., Ruan R.R., 2009 Cultivation of green algae Chlorella sp. in different wastewaters from municipal wastewater treatment plant. Applied Biochemstry and Biotechnology. doi:10.1007/s12010-009-8866-7.
- Feng Y., Li Chao., Zhang D., 2011 Lipid production of Chlorella vulgaris cultured in artificial wastewater medium. Bioresource Technology; 101: 101–105.
- Yujie F., Li C., Zhang D., 2011 Lipid production of Chlorella vulgaris cultured in artificial wastewater medium. Bioresource Technology; 102: 101–105.
- Kong Q.X., Li L., Martinez B., Chen P., Ruan R., 2010 Culture of microalgae Chlamydomonas reinhardtii in wastewater for biomass feedstock production. Applied. Biochemistry Biotechnology; 160: 9–18.
- Bligh, E.G., Dyer, W.J., 1959 A rapid method of total lipid extraction and purification. Canadian Journal of Biochemical Physiology; 37, 911–917.
- Knothe G., 2006 “Analyzing Biodiesel: Standards and other Methods.” Journal of the American Oil Chemists’ Society 83; 10: 823–833.
- Ramachandra T. V., Alakananda B. and Supriya G., 2011, Biofuel prospects of microalgal community in urban wetlands, International Journal of Environmental Protection (IJEP).1( 2):.54-61
- Brune D.E., Lundquist T.J., Benemann J.R., 2009 Microalgal biomass for greenhouse gas reductions: potential for replacement of fossil fuels and animal feeds. Journal of Environmental Engineering; 135: 1136–1144.
|
|
Citation : T.V. Ramachandra and Durga Madhab Mahapatra, 2012. Scope for Algal Biofuel from Wastewater., Proceedings of the 8th National Conference on Indian Energy Sector, Synergy with Energy, AMA, Ahmedabad. October 11-12, 2012, Pp. 72–79.
|